Between the gas phase and the condensed phase of liquids and solids, lies an intermediate state of matter that is not quite one phase or the other. Called clusters, these small agglomerations of atoms and molecules have properties not found in gases or condensed matter. This relatively new fields of cluster science has the potential to make important advances in areas as far reaching as materials science, environmental chemistry, catalysis and biochemistry.
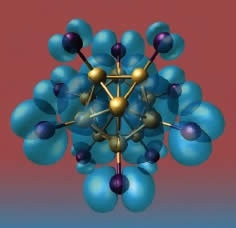
Figure 1. Simulation of a superatom
Initial Discoveries
Will Castleman, a chemistry and physics professor at Penn State, has been studying this intermediate state of matter since it was first recognized in the early 1960s. "It started with those of us, in my case, using mass spectrometers to study unique reactions related to the behavior of radioactive materials. I worked for Brookhaven National Laboratory at the time. It was before I even had my Ph.D., but I was running a research group that was looking at radioactive materials in relation to their behavior in possible nuclear accidents. We discovered, looking through the mass spectrometer, that many of the materials were clusters."
Intermediate Phase Materials
At first they believed that these clusters were small pieces of a condensed phase. But soon they realized that clusters were an intermediate phase, often with different structures and properties from the bulk material. Castleman and the other pioneers of cluster science hoped that by studying this new phase of matter, they could learn how matter changed from gasses into liquids and solids. "Since then, my group has been working with larger and larger clusters, studying reactivity, bonding, structure, and spectroscopy changes, and we've been able to bridge these fields by working our way through the intermediates to learn more about the condensed phase. Along the way we've learned there are things in between the phases that are as interesting if not more so than the phases themselves."
Metal and Carbon Combine to Form New Material Structure
It was in Castleman's labs in the 1990s that a novel class of nanoclusters was discovered that opened a new field of materials research. Made up of molecules with cage-like structures of transition metals bound to carbon atoms, these metallo-carbohedrenes, or Met-Cars, have potential uses as catalysts and unique electronic and optical materials.
Met-Cars, Metallo-Carbohedrenes
Like many scientific discoveries, Met-Cars were stumbled upon in the process of looking for something else. While studying a series of catalytic reactions, two of Castleman's graduate students, Baochuan Guo and Kevin Kerns, turned their laser onto a rod of titanium over which an ethylene gas was flowing. They passed the small quantity of material they produced through the mass spectrometer. An unusually strong peak occurred at a single spot, showing a mass of 528. After ruling out several possibilities, Castleman feared the peak was an artifact, a bit of dirt in the equipment. He had his assistants take the equipment apart and clean it, but when they ran the experiment again the peak remained. It turned out to be a stable cluster of 12 carbon and 8 titanium atoms.
Met-Car Properties
One of the unusual properties of metallo-carbohedrenes is associated with their ionization potential, the amount of energy it takes to remove an electron from a system. For each of the transition metals, the ionization potential is fairly high, around 8 electron volts. For the carbon atoms, the potential is even higher, around 12.5 electron volts. However, when metal and carbon atoms are combined in this unique structure, the ionization potential drops down to about 4 electron volts. In this respect, Met-Cars behave similarly to the highly reactive alkali metals on the far left side of the periodic table. "That came as a big surprise to us. It suggested that Met-Cars could have some interesting semiconductor properties. This makes them good model systems to understand more about electrons in metals and electrons in confined systems."
A Bowlful of Atoms
Castleman's group began assembling various materials into larger clusters and found some surprising results. Some clusters of atoms began to mimic the behavior of different atoms on the periodic table. "I thought, ‘Wow, these are real super atoms,' and I guess it caught on. We thought it was an appropriate name, and most people are now calling them superatoms."
Magic Numbers of Atoms
While working with clusters of aluminum atoms, his group noticed that when they attached an electron to a particular cluster with 13 aluminum atoms, the cluster did not react at all - unusual behavior for aluminum, which is typically so reactive that aluminum storm windows will turn whitish due to an oxidized surface. But aluminum 13 with an extra electron did not oxidize at all. The same was true with aluminum 23 and aluminum 37, but not the rest of the clusters. "We called these magic numbers, because those clusters seemed like magic."
Jellium Model
To understand why some clusters of a certain number of atoms reacted so differently from the rest, Castleman turned to what scientists have called the jellium model. This model predicts that atoms in a confined system will share their free electrons, filling out the available orbitals, the electron shells, of the entire group of atoms. "Think of it as jelly. You don't have to worry where the individual atoms are, they're all held together in a bowl. You can feed electrons into the jelly and see how the energy builds up."
Energy Levels
Energy levels in bulk materials are significantly different from materials in the nanoscale. Castleman, who has a flair for analogy, puts it this way: "Adding energy to a confined system such as a cluster is like putting a tiger in a cage. A tiger in a big zoo with open fields will act more relaxed, because he has a lot of room to wander around. If you now confine him in smaller and smaller areas, he gets nervous and agitated. It's a lot that way with electrons. If they're free to move all around through a metal, they have low energy. Put them together in a cluster and shine light on them, they get very excited and try to get out of the structure. A big part of our work is with lasers, to initiate the formation of the clusters and then to characterize the clusters. Lasers and mass spectrometers are our primary tools."
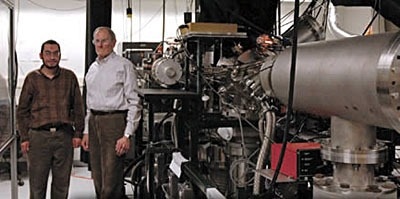
Figure 2. A. Welford Castleman (r) with graduate student Mohamed Sobhy
Building the Superatom
The Castleman group, which currently numbers 20 Ph.D. students, works on various problems that have long-term implications for understanding matter, but which have few immediate pay-offs, as well as other problems with more immediate benefits, such as atmospheric and environmental research. Their research has expanded into half a dozen large laboratory spaces that are a jungle of power cables, crisscrossing tubes, and table-size machines, such as ultrafast lasers, molecular beams, and flow reactors.
"Our apparatus are not commercial in any sense of the word," Castleman says, as we pick our way through the labyrinth of labs and come unexpectedly on students deeply engrossed in experiments. "All of the apparatus we use in our labs, with the exception of one, were designed by us and built in our shops. The students do some of the actual building. The apparatus are usually undergoing rebuilding monthly if not weekly, because we are always reconfiguring to accommodate the next experiment that looks like an exciting problem to pursue. It is a fantastic experience for my students. They learn sophisticated electronics and sophisticated computer programming. They teach each other and interact with a lot of the staff in our machine and electronics shops."
Femtosecond Lasers
Along with sophisticated mass spectrometers, the students use ultrafast femtosecond lasers to understand the dynamics of clusters. Castleman provided another analogy to illustrate the concept of a femtosecond: "A femtosecond is 10 to the minus 15 seconds, an incredibly small period of time. It's hard to visualize so small a time, but being from Penn State, I imagine a football field covered three feet deep in beach sand. Imagine counting all the grains of sand in that football field, plus 25 other football fields, and that ends up being about 10 to the 15 grains of sand. We typically work at 50 to 100 femtoseconds. You can actually watch atoms and electrons moving around and see materials respond to optical excitation. In this way we can learn a lot about the properties of the matter we're creating."
Nanoscale Material Building Blocks
Toward the end of 2006, Castleman and his colleagues, Ayusman Sen and Paul Weiss in Chemistry, and a collaborator at Virginia Commonwealth University, theoretical physicist Shiv Khanna, received a large Multidisciplinary University Research Initiative (MURI) grant to study cluster assembled materials as possible building blocks for nanoscale materials. "Clusters are potentially better for building nanomaterials than building one atom at a time, because you can better control individual properties. If the superatoms retain their structure, then in principle the materials you are putting together should maintain their properties. That has to be proved for each individual system."
One of the first focuses of their research will be to see if they can take their old friend Aluminum 13, and use it to improve the performance of rocket fuel. Aluminum particles impart a tremendous boost to fuels, but tend to react with oxygen quickly in storage. A non-reactive Al13 cluster, bound to the right organic molecule, could solve the storage problem.
Rewriting the Periodic Table
Extending the periodic table to a new group of superatoms is one of the possibilities of cluster science that created both excitement and trepidation among chemists and physicists, not to mention high school chemistry students.
"It may be a little tongue in cheek to say that we are rewriting the periodic table, but what we are doing is finding clusters that really do have in some sense properties that resemble all of the atoms in the periodic table. For instance, Al13- reacts like a rare gas. For all intents and purposes you would think it was a rare gas. We found other clusters that behave like a halogen. Others like an alkaline earth metal. And others like multivalent atoms of the periodic table. Now they don't behave exactly alike because of different numbers of neutrons and protons, but chemically they behave very much like atoms of the periodic table. The exciting thing has been we've discovered some of these that retain their properties and their structures when we put them together with something else. That is the most promising aspect of this field. There is a real good chance of being able to tailor design a lot of materials. Not every material, obviously, but there is a lot of promise in this superatom concept."
|