Nanoparticles were first developed approximately 35 years ago [1]. They were initially developed as carriers for vaccines and cancer chemotherapy agents.
In delivery of any nanoparticle inside the body pharmacokinetics and pharmacodynamics play a very important role. Pharmacokinetics deals with drug delivery inside the human body. When a drug inters the body intravenously it goes through absorption, distribution, metabolism & elimination. The result depends mainly on the physiochemical properties of the drug (Molecular weight, shape, charge, and aqueous solubility) and therefore on its chemical structure. The target of all complicated drug delivery systems, therefore, is to deliver medications to specifically targeted parts of the body through a medium that can control the therapy’s administration by means of either a physiological or chemical trigger. A successful drug carrier system needs to demonstrate optimal drug loading and release properties, long shelf-life and low toxicity. Nanoparticles are desirable for drug delivery because of a number of properties. They can be used to increase drug solubility, have lower toxicity, provide a drug direct, increase bioavailability and target drug delivery [2].
In the first part of the paper we focused on nanoparticles, their transport & cancer drug delivery. In the second part we discuss biosensors ,their relevance in health care and in the last part, development of nanobiosensors, their role in cancer care, biosensors and the global scenario.
In the past few years scientists have shown enough interest for successful drug delivery using nanoparticles. New era is more promising because scientists have their latest development i.e. biosensors/nanobiosensors. Biosensors have been under development [3] for over 35 years and research in this field has become very popular for 15 years. Although biosensors are used for several clinical applications, few biosensors have been developed for cancer-related clinical testing. It is important to understand the complexity and some of the basic elements of cancer biology when developing biosensors for cancer testing. The use of biosensors for cancer clinical testing may increase assay speed and flexibility, enable multi-target analyses and automation and reduced costs of diagnostic testing. It has shown great potential to eradicate many diseases including cancer.
Overall this paper sheds light on latest developments in the field of cancer care.
Cancer and Drug Delivery
Cancer is an uncontrolled disease but its fate is still undecided. No doubt in the last few decades scientists have shown the progress to defeat this disease but are still not able to eradicate cancer from society.
Table 1. Tumour Type
Vascular Tumor
|
50% Cells
|
Before blood vessels
|
10% Blood vessels
|
Nutrient obtained via diffusion
|
40% Extra cellular matrix
|
Cells in the center starve
|
Vessel structure is not organized
|
Cells on the periphery increase
|
May be benign and encapsulated or malignant
|
Cells in the interior are quiescent
|
|
Growth limited to a few mm in diameter
|
Tumor blood vessels have several abnormalities compared with physiological vessels like relatively high proportion of proliferating endothelium cells, an increased tortuosity and an aberrant basement membrane formation. The rapidly increasing tumor vasculature often has discontinuous endothelium, with gaps between the cells that may be several hundred nanometres large [4].
Macromolecular transport pathways across tumor vessels occur via open gaps (Interendothelial junctions and transendothelial channels), vesicular vacuolar organelles and fenestrations. However it remains controversial which pathways are predominantly responsible for hyperpermeability and macromolecular transvascular transport.
Tumor interstitium is also characterized by a high interstitial pressure, leading to an outward convective interstitial fluid flow, as well as the absence of an anatomically well defined functioning lymphatic network. Hence, the transport of an anticancer drug in the interstitium will be governed by physiological (i.e., Pressure) and physicochemical (i.e., composition, structure) properties of the interstitium and by the physiochemical properties [5] of the molecules itself (i.e., size, configuration, charge, hydrophobicity). Physiological barriers at the tumor level (i.e., poorly vascularized tumor regions, acidic environment, high interstitial pressure and low microvascular pressure) as well as the cellular level (i.e., altered activity of specific enzyme systems, altered apoptosis regulation and transport based mechanism) and in the body (i.e., distribution, biotransformation and clearance of anticancer agent) must be overcome to delivery anticancer agents to tumor cells in vivo.
A strategy could be to associate antitumor drugs with colloidal nanoparticles, with the aim to overcome non-cellular and cellular based mechanisms of resistance and to increase selectivity of drugs towards cancer cells while reducing their toxicity towards normal tissues. Very slow, time-consuming development in the treatment of severe diseases has led to the adoption of a multidisciplinary approach to the targeted delivery and release of drugs, underpinned by nanoscience and nanotechnology.
Drug carriers include micro and nanoparticles, micro and nanocapsules, lipoproteins, liposomes, and micelles, which can be engineered to slowly degrade, react to stimuli and be site-specific. The ultimate aim is to minimize drug degradation and loss, prevent harmful side effects and increase the availability of the drug at the disease site.
Chemotherapy via Nanoparticles
Chemotherapy using nanoparticles [6] has been studied in clinical trials for several years and lots of studies have been published in this regards. In general, nanoscale drug delivery systems for chemotherapy can be divided into two categories: Polymer and lipid based. Polymer based nanoparticles are more successful.
Nanoparticles and Their Role in Cancer
Nanoparticles play a very important role in cancer research. Due to extremely small size of nanomaterials they are more readily taken up by the human body. Nanomaterials are able to cross biological membranes and access cells, tissues and organs that larger-sized particles normally cannot. Nanoparticles are stable, solid colloidal particles consisting of biodegradable polymer [7] or lipid materials and range in size from 10 to 1,000 nm. Drugs can be absorbed onto the particle surface, entrapped inside the polymer / lipid, or dissolved within the particle matrix.
Nanotechnology has tremendous potential to make an important contribution in cancer prevention, detection, diagnosis, imaging and treatment. It can target a tumor, carry imaging capability to document the presence of tumor, sense pathophysiological defects in tumor cells, deliver therapeutic genes or drugs based on tumor characteristics, respond to external triggers to release the agent and document the tumor response and identify residual tumor cells.
Nanoparticles have benefits because of its size. Because of their size they can easily enter small places. Nanoparticles have attracted the attention of scientists because of their multifunctional character. Nanoparticles have large surface area to volume ratio, that helps in diffusion [8] also leading to special properties such as increased heat and chemical resistance.
Transport of Oxygen, Nutrient & Nanoparticles Inside Tumor
Most of the ~1013 cells in the human body [9] are within a few cell diameters of a blood vessel. This remarkable feat of organization facilitates the delivery of oxygen and nutrients to the cells that form the tissue of the body. It also enables the efficient delivery of most medicines. As a result of poorly organized vasculature in solid tumors, there is limited delivery of oxygen and other nutrients to cells that are distant from functional blood vessels.
A single cancerous cell [10] enclosed by healthy tissue will replicate at a rate higher than the other cells, placing a strain on the nutrient supply and removal of metabolic waste products. Once a small tumor mass has formed, the healthy tissue will not be able to fight with the cancer cells for the inadequate supply of nutrients from the blood stream. Tumor cells will move healthy cells until the tumor reaches a diffusion- limited maximal size. While tumor cells will typically not begin apoptosis in a low nutrient environment, they do require the normal building blocks of cell function like oxygen, glucose and amino acids. The vasculature was designed to supply the now extinct healthy tissue that did not place as high a demand for nutrients due to its slower growth rate. Tumor cells will therefore continue dividing because they do so without regard to nutrient supply but also many tumor cells will die because the amount of nutrients is not enough. The tumor cells at the outer edge of a mass have the best access to nutrients while cells on the inside die creating a necrotic core within tumors that rely on diffusion to deliver nutrients and remove waste products.
In essence, a steady state tumor size forms, as the rate of proliferation is equal to the rate of cell death until a better connection with the circulatory system is created.
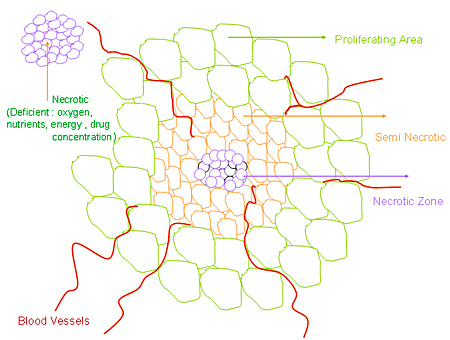
Figure 1. Cancer tumor: Necrotic, Seminecrotic, Proliferating area
Active tumor targeting of nanoparticles [11] involves attaching molecules, known collectively as ligands, to the outsides of nanoparticles. These ligands are extraordinary in that they can recognize and bind to complementary molecules, or receptors, found on the surface of tumor cells. When such targeting molecules are added to a drug delivery nanoparticle, more of the anticancer drug finds and enters the tumor cell, increasing the efficiency of the treatment and reducing toxic effects on surrounding normal tissue. Different types of nanoparticles are developed to deliver drug at target site.
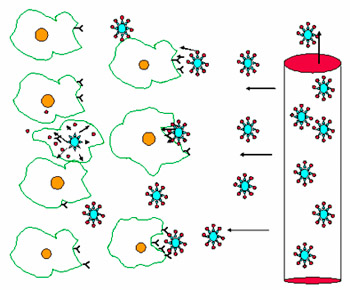
Figure 2. The ligands on the surface of the nanoparticles fit into the cell receptors, allowing encapsulated drug molecules to enter the tumor cell after binding
Nanoparticles and other nanostructures appear to hold great promise for the future of cancer treatment. Characteristics of nanoparticles [8] for cancer drug delivery are given below:
Table 2. Characteristics of nanoparticles used for cancer drug delivery
|
Carbon magnetic Nanoparticles
|
40-50 nm
|
For drug delivery and targeted cell destruction
|
Dendrimers
|
1-20 nm
|
Holding therapeutics substances such as DNA in their cavities
|
Ceramics Nanoparticles
|
~35 nm
|
Accumulate exclusively in the tumor tissue and allow the drug to act as sensitizer for photodynamics therapy without being released
|
Chitosan Nanoparticles
|
110-180 nm
|
High encapsulation efficiency .In vitro release studies show a burst effect flowed by a slow and continuous release.
|
Liposomes
|
20-25 nm
|
A new generation of liposomes that incorporate fullerenes to deliver drug that are not water soluble, that tend to have large molecules
|
Low Density Lipoprotein
|
20-25 nm
|
Drug solublized in the lipid core or attached to the surface
|
Nanoemulsions
|
20-25 nm
|
Drug in oil/or in liquid phases to improve absorption
|
Nanolipispheres
|
25-50 nm
|
Carrier incorporation of lipophilic and hydrophilic drugs
|
Nanoparticles composites
|
~40 nm
|
Attached to guiding molecules such as Mabs for targeted drug delivery
|
Nanoparticles
|
25-200 nm
|
Act as continuous matrices containing dispersed or dissolved drug
|
Nanopill/Micelle
|
20-45 nm
|
Made for two polymer molecules-one water -repellent and the other hydrophobic-that self assemble into a sphere called a micelle that can deliver drugs to specific structures within the cell
|
Nanospheres
|
50-500 nm
|
Hollow ceramic nanospheres created by ultrasound
|
Nanovesicles
|
25-3000 nm
|
Single or multilamellar bilayer spheres containing the drugs in lipids
|
Polymer Nanocapsules
|
50-200 nm
|
Used for enclosing drugs
|
Biosensors, its History & Relevant Applications
Biosensors play a vital and important role in medicine, industry and the environment, providing routine analysis, crucial monitoring, and early detection of problems and crisis points.
What Are Biosensors?
A biosensor is a device for the detection of an analyte that combines a biological component with a physicochemical detector component. It combines a biochemical recognition/binding element (ligand) with a signal conversion unit (transducer).
Biosensor

Analyte

Bio-receptor

Transducer

Biocatalyst

The output from the transducer is amplified, processed and displayed
Figure 3. Schematic flow chart showing the main components of a biosensor.
The history of biosensors [12] is very old. In 1956, Leland C Clark Jr., who is known as the father of Biosensors, published his definitive paper on the oxygen electrode.
Table 3. Defining events in the history of biosensor development [13]
|
1916
|
First report on the immobilization of proteins: adsorption of invertase on activated charcoal
|
1922
|
First glass pH electrode
|
1956
|
Invention of the oxygen electrode
|
1962
|
First description of a biosensor: an amperometric enzyme electrode for glucose
|
1969
|
First potentiometric biosensor: urease immobilized on an ammonia electrode to detect urea
|
1970
|
Invention of the Ion-Selective Field-Effect Transistor (ISFET)
|
1972/5
|
First commercial biosensor: Yellow Springs Instruments glucose biosensor
|
1976
|
First bedside artificial pancreas (Miles)
|
1980
|
First fibre optic pH sensor for in vivo blood gases
|
1982
|
First fibre optic-based biosensor for glucose
|
1983
|
First surface plasmon resonance (SPR) immunosensor
|
1984
|
First mediated amperometric biosensor: ferrocene used with glucose oxidize for the detection of glucose
|
1987
|
Launch of the MediSense ExacTech™ blood glucose biosensor
|
1990
|
Launch of the Pharmacia BIACore SPR-based biosensor system
|
1992
|
i-STAT launches hand-held blood analyzer
|
1996
|
Glucocard launched
|
1996
|
Abbott acquires MediSense for $867 million
|
1998
|
Launch of LifeScan Fast Take blood glucose biosensor
|
1998
|
Merger of Roche and Boehringer Mannheim to form Roche Diagnostics
|
2001
|
LifeScan purchases Inverness Medical's glucose testing business for $1.3billion
|
2003
|
i-STAT acquired by Abbott for $392 million
|
2004
|
Abbott acquires TheraSense for $1.2 billion
|
Biosensor Components
Biosensor consists of three parts [14]:
• The sensitive biological element (e.g. Tissues, Microorganisms, Organelles, Cell receptors, Enzymes, Antibodies, Nucleic acids, Synthetic receptors, Sensing organs etc)
• The transducer (Acts as an interface, measuring the physical change that occurs with the reaction at the bioreceptor then transforming that energy into measurable electrical output.) Physical transducers: Optical, Electrochemical, Opto-electronic, Piezoelectric, Magnetic Thermal, Mass.
• The detector element (Signals from the transducer are passed to a microprocessor where they are amplified and analyzed, The data is then converted to concentration units and transferred to a display or/and data storage device.)
The transducer [15] converts the biochemical interactions into a measurable electronic signal. Electrochemical, electrooptical, acoustical and mechanical transducers are among the many types found in biosensors. The transducer works either directly or indirectly.
Table 4. Principal of detection
|
Piezoelectric
|
Mass
|
Electrochemical
|
Electric distribution
|
Optical
|
Light intensity
|
Calorimetric
|
Heat
|
Direct Detection Biosensors
Direct recognition sensors, in which the biological interaction is directly measured typically, use non-catalytic ligands such as cell receptors or antibodies.
Indirect Detection Biosensors
The second class of transducers, indirect detection sensors, relies on secondary labelled elements that are often fluorescently tagged antibodies or catalytic elements such as enzymes.
Biosensors are also characterized [16] by their specificity, or their ability to recognize a single compound among other substances in the same sample. The selectivity of biosensors is determined by both the bioreceptor and the method of transduction.
There are many potential applications of biosensors. The main requirements for a biosensor approach to be valuable in terms of research and commercial applications.
Few relevant applications are given below:
• Cancer diagnostics
• Detection of pathogens
• Drug discovery and evaluation of biological activity of new compounds
• Determination of drug residues in food such as antibiotics
• Glucose monitoring in diabetes patients etc.
Table 5. Different biosensors, principal, applications and relevant references
|
Optical biosensor
|
Colorimetric for color, Photometric for light intensity
|
Detection of disease like cancer, direct glucose determination in blood using reagentless optical biosensor
|
[17], [18]
|
Surface Plasmon resonance (SPR)* biosensor (optical biosensor) (Invented: Biacore International AB (Biacore), 1990 )
|
Evanescent wave phenomenon
|
Detection and identification of biological analytes and biophysical analysis of biomolecular interactions
|
[19]
|
Electrochemical biosensor
|
Immobilization of biomolecules
|
Use in Bioaffinity Assays for Determining DNA and Its Effectors, also Electrochemical measurement of blood glucose concentrations
|
[20], [21]
|
DNA biosensors
|
Nucleic acid hybridization rennealing between the ssDNAs from different sources
|
DNA biosensors can detect the presence of genes or mutant genes associated with inherited human diseases
|
[22]
|
Nanotube based biosensor (Carbon nanotubes (CNTs) discovery by Iijima in 1991)
|
We report a system for bimolecular assays that uses carbon nanotubes as both an electrode and an immobilization phase in an electrochemiluminescence based sensing device
|
Detection of bio- molecules like Streptavidin and IgG, application in immunoassays, nuclic acid probe assays, assays for clinical chemistry analytes
|
[23], [24]
|
Piezoelectric biosensor
|
based on nucleic acids interaction/DNA based
|
To point mutation detection in PCR samples.
|
[26]
|
* The principle of SPR is based on the evanescent wave phenomenon, discovered by Wood in 1902.
Other optical biosensors are mainly based on changes in absorbance or fluorescence of an appropriate indicator compound.
Application [27] of biosensors in cancer clinical testing has several potential advantages over other clinical analysis methods including increased assay speed and flexibility, capability for multi-target analyses, automation, reduced costs of diagnostic testing and a potential to bring molecular diagnostic assays to community health care systems and to underserved populations.
Biosensors have several potential advantages [21] over other methods of cancer analysis, especially increased assay speed and flexibility. Rapid, real-time analysis can provide immediate interactive information to health care providers that can be incorporated into the planning of patient care. In addition, biosensors allow multi-target analyses, automation, and reduced costs of testing. Biosensor-based diagnostics might facilitate cancer screening and improve the rates of earlier detection and attendant improved prognosis. Such technology may be extremely useful for enhancing health care delivery in the community setting and to underserved populations.
Biosensor-based diagnostics might facilitate cancer screening and improve the rates of earlier detection and attendant improved prognosis. Such technology may be extremely useful for enhancing health care delivery in the community.
Development of Nanobiosensors
One of the most recent technological advances in this area has been the development of nanosensors, which are sensors with dimensions on the nanometer scale. Nanosensors of various have been reported in the literature over the past decade.
The emergence of nanotechnology is opening new horizons for the development of nanosensors and nanoprobes with submicron-sized dimensions that are suitable for intracellular measurements.
Various readout techniques - optical, electrochemical, or mass-sensitive - can be used for detection in biosensors.
Also there are currently several hypothesized ways to produce nanosensors:
• Top-Down Method
• Bottom-up Method
• Self-assembly
Biosensor/ Nanobiosensor (Monitoring Environmental Health at the Single Cell Level)
Nanomaterials are exquisitely sensitive chemical and biological sensors. Each sensor [28] should be sensitive for one chemical or biological component of a substance. Thus, by having sensor arrays it is possible to tell the composition of an unknown substance.
The application area will be wide, encompassing food industry, detection of pollution, medical sector, brewery etc. A biosensor generally consists of a biosensitive layer that can either contain biological recognition elements or be made of biological recognition elements covalently attached to the transducer. The interaction between the target analyte and the bioreceptor is designed to produce a physicochemical perturbation that can be converted into a measurable effect such as an electrical signal. Bioreceptors are important elements providing specificity for biosensor technologies, because they allow for binding of the specific analyte of interest to the sensor for the measurement with minimum interference from other components in complex sampling mixtures. Biological sensing elements can be either a biological molecular species (eg, an antibody, an enzyme, a protein, or a nucleic acid) or a living biological system (eg, cells, tissue, or whole organisms) that uses a biochemical mechanism for recognition.
A nanobiosensor [29] also referred to a nanosensor, is a biosensor with dimensions on the nanometer scale (1 nm = 10–9 m). So, Nanosensors are any biological, chemical, or physical sensory points used to convey information about nanoparticles to the macroscopic world. Though humans have not yet been able to synthesize nanosensors, predictions for their use mainly include various medicinal purposes and as gateways to building other nanoproducts, such as computer chips [30] that work at the nanoscale and nanorobots.
Presently, there are several ways proposed to make nanosensors, including top-down lithography, bottom-up assembly, and molecular self-assembly.
Importance of Nanosensors in Cell Analysis
• Cells in a population respond asynchronously to external stimuli e.g., apoptosis, cells differ in their ability to activate caspases
• To study and understand molecular mechanisms that underlie such differences it is necessary to measure caspase activity in individual cells
Nanomaterials are exquisitely sensitive chemical and biological sensors. Nanosensors with immobilized bioreceptor probes that are selective for target analyte molecules are called nanobiosensors. Nanosensors offer significant improvements [31] in the filed of cancer research. There are many developed biosensors and nanobiosensors, few of them are given below:
Optical Biosensor
A sensor that uses light to detect the effect of a chemical on a biological system is an optical biosensor. The construction and use of optical nanosensors was first reported by Kopelman et al. in 1992. Optical nanosensors [32], like larger sensors, can typically be classified into one of two wide categories, chemical or biological, depending on the probe used. Both types of sensor have been used to offer a reliable method of monitoring various chemicals in microscopic environments and have even been used to detect different entities within single cells.
Latest developments in nanotechnology have led to the development of optical nanosensor systems [33] whose nanoscale dimensions are suitable for intracellular measurements.
Electrical Nanosensors
Electrical nanosensors incorporating nanostructures as sensing probes are highly promising devices for disease diagnostics in medicine; chemical or biomolecular binding events are directly converted into electrical signals, which allows for label-free readout in the case of DNA detection.
Electrochemical Biosensor
Nanomaterials are acquiring a big impact on progress of electrochemical biosensors [34]. Nanotechnology brings new possibilities for biosensors construction and for developing novel electrochemical bioassays. The use of nanoscale materials for electrochemical biosensing has seen explosive growth in the past 5 years. Nanoscale materials have been used to achieve direct wiring of enzymes to electrode surface, to promote electrochemical reaction, to impose nanobarcode for biomaterials and to amplify signal of biorecognition event.
The electrochemical nanobiosensors are applied in areas of cancer diagnostics and detection of infectious organisms.
Such devices will develop toward reliable point-of-care diagnostics of cancer and other diseases, and as tools [35] for intra-operation pathological testing, proteomics and systems biology.
In-body electronic nanosensors may offer understanding of dynamic processes in cells and new treatments for disease.
Nanowire Biosensor
Nanowire based biosensor [36] arrays have significant impact for detection of biological threats, early diagnosis of cancer, drug discovery, and medical treatment. The nanowire based biosensor arrays enables simultaneous detection of multiple analytes such as cancer biomarkers in a single chip, as well as fundamental kinetic studies for biomolecular reactions.
Viral Nanosensor
Virus particles [37] are essentially biological nanoparticles. Herpes simplex virus (HSV) and adenovirus have been used to trigger the assembly of magnetic nanobeads as a nanosensor for clinically relevant viruses. This system is more sensitive than ELISA-based methods and is an improvement over PCR-based detection because it is cheaper, faster and has fewer artifacts.
Nanoshell Biosensors
Gold nanoshells [38] have been used in a rapid immunoassay capable of detecting analyte within complex biological media without any sample preparation. Aggregation of antibody/nanoshell conjugates with extinction spectra in the near infrared is monitored spectroscopically in the presence of analyte. Nanoshells are already being developed for applications including cancer diagnosis, cancer therapy, diagnosis and testing for proteins associated with Alzheimer's disease.
Nanotube Based Biosensors
This is a single wall carbon nanotube (SWNT) based biological sensor [23] for the detection of bio molecules. By coating the surfaces of tiny carbon nanotubes with monoclonal antibodies, biochemists and engineers at Jefferson Medical College and the University of Delaware have teamed up to detect cancer cells in a tiny drop of water.
This approach is used towards developing a biosensor for breast cancer detection [39], by functionalizing the CNTs with antibodies that are specific to cell surface receptors of breast cancer cells.
Nanobiosensors and Cancer
With the progress of biosensor technology, the range of applications expands. Numerous biosensor applications for cancer diagnostics are described. Nanobiosensor plays very important role in cancer care. Bio-conjugated particles [40] and devices are also under progress for early cancer detection in body fluids such as blood and serum. These nanoscale devices operate on the principles of selectively capturing cancer cells or target proteins. The sensors are often coated with a cancer-specific antibody or other biorecognition ligands so that the capture of a cancer cell or target protein yields an electrical, mechanical, or optical signal for detection. For example, microelectrical mechanical systems (MEMS) sensors rely on the deflection of nanometer-scale cantilever beams such as carbon nanotubes and metallic oxide nanobelts, structures that are sensitive to piconewton mechanical forces. New nanosensor uses quantum dots to detect DNA. Using tiny semiconductor crystals [41], biological probes and a laser, Johns Hopkins University engineers have developed a new method of finding specific sequences of DNA by making them light up beneath a microscope. The researchers, who say the technique will have important uses in medical research, demonstrated its possibility in their lab by detecting a sample of DNA containing a mutation linked to ovarian cancer.
The "Nanobiosensor" allow scientists to physically probe inside a living cell without destroying it. As scientists adopt a systems approach to studying biomolecular processes, the nanobiosensor provides a valuable tool for intracellular studies that have applications ranging from medicine to national security to energy production. Professor Calum McNeil at Newcastle University and his work group are developing a tiny biosensor to detect cancer proteins and potentially the bug that causes MRSA (Meticillin-resistant Staphylococcus aureus). The device would work by identifying cancer markers - proteins or other molecules produced by cancer cells. These vary according to the type of cancer and are distinct from proteins produced by healthy cells. It may possible research towards this direction for cancer treatment come with successful outcome.
The nanobiosensor has many other applications for looking at how cells react when they are treated with a drug or invaded by a biological pathogen. This has important implications ranging from drug therapy development to national security, environmental protection and a better understanding of molecular biology at a systems level.
Point of Care Testing
One of the most hopeful applications of biosensors is for point-of-care testing (POCT); diagnostic testing that is performed on site. POCT offers the potential for faster and cheaper diagnostics.
Identification of Biomarkers

Validation of Cancer Biomarkers

Cancer Biomarkers

Ligands / Probes Developments

Cancer Diagnostics Biosensor Detector

Point of Care Cancer Diagnostics
Figure 4. The basic components required for using biosensors in clinical cancer diagnosis
Cancer biomarkers identified from basic and clinical research, and from genomic and proteomic analyses must be validated. Ligands and probes for these markers can then be combined with detectors to produce biosensors for cancer-related clinical testing. Point of Care cancer testing requires integration and automation of the technology as well as development of suitable sample preparation methods. Biosensor-based diagnostics [21] might make easy cancer screening and get better the rates of earlier detection and attendant improved prognosis. Such technology may be extremely helpful for enhancing health care delivery in the community setting and to underserved populations. Point of care testing is beneficial for cancer care but at the same times many challenges (development of reproducible biomarker assays; development of multi-channel biosensors; advances in sample preparation and cancer cell enrichment; development of new, more sensitive transducers, advanced manufacturing techniques etc.) are still presented.
Global Scenario
Since the first commercialization of biosensors in 1991, there has been an exponential increase in the literature that has dealt with new biosensors and their various applications. Many biosensors are in the market now days which is based on latest technology. Nanosensor arrays [42] are already under development by giant firms in healthcare Industries. Nano Markets foresees important applications for nanosensors in a wide range of applications including medicine and healthcare. Sensors designed and built using nanotechnology will generate global revenues of $2.7 billion in 2008 and reach $17.2 billion in 2012 and Nano Markets expects the market for biomedical nanosensors to reach approximately $800 million in 2008 and $1.2 billion in 2012.
Nanosensors will better detect the onset of diseases such as cancer or heart disease. Consequently, nanosensors [16] have yet to be made compatible with most consumer technologies for which they have been projected to eventually enhance. Biosensors represent a rapidly expanding field, at the present time, with an estimated 60% annual growth rate; the major impetus coming from the health-care industry (e.g. 6% of the western world are diabetic and would benefit from the availability of a rapid, accurate and simple biosensor for glucose) but with some pressure from other areas, such as food quality appraisal and environmental monitoring.
The estimated world analytical market is about £12,000,000,000 year of which 30% is in the health care area. There is clearly a vast market expansion potential as less than 0.1% of this market is currently using biosensors.
Conclusion
Biosensors offer an innovative, but under-utilized technology. This paper reviewed recent progress in research on nanotechnology and cancer. The development of nanosensors has already had a large impact on biological and biomedical research.
With the growing interest in and practical use of nanotechnology, the function of nanosensors to give different types of cellular measurements is rising speedily. The possibilities provided by nanosensor technology have begun and will continue to change the field of cellular biology/cancer biology. Still more accurate protocol is required to improve the cancer research. It is quite likely that nanotechnology will become the next frontier of medical research.
Acknowledgment
The work is supported by Department of Science and Technology (DST), New Delhi, India. Authors are very grateful for the hospitality of the Indian Institute of Technology Roorkee.
|