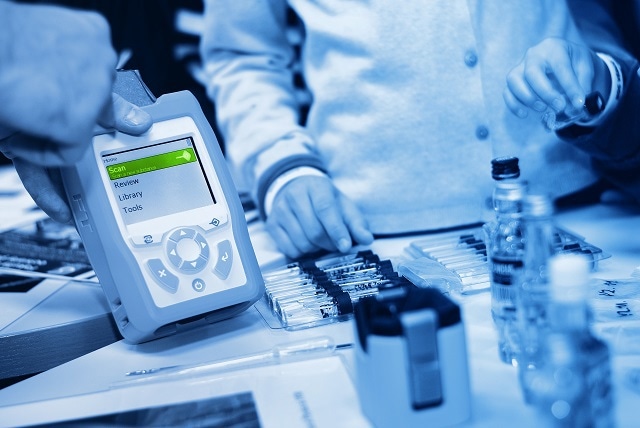
Image Credits: Forance/shutterstock.com
Spectroscopy is a technique widely used in analytical chemistry to identify and characterise samples of material.
The technique is based on the fact every atom interacts with electromagnetic radiation in a unique way to produce its own fingerprint identity.
Raman spectroscopy is a type of vibrational spectroscopy like the more widely used infrared spectroscopy. It measures the way light is scattered by a material. In most cases the wavelength of the scattered light is identical to the incident light and is known as Rayleigh scattering. But in a few cases energy is lost or gained when the light is scattered, so it has a different wavelength to the incident light. This is known as Raman scattering.
Raman Spectroscopy is useful because it gives detailed structural information without being destructive and only needs a minimal amount of sample.
Surface-Enhanced Raman Spectroscopy
The major drawback of Raman spectroscopy is the fact that the Raman scattering effect is weak. Only about one in 10 million scattered photons are Raman scattered.
Surface-Enhanced Raman Spectroscopy (SERS) dates back to 1977 and has so far produced the greatest amplification of the weak signal. It typically enhances the intensity of the Raman signal by factors of 104 to 106.
Raman scattering is highly informative but suffers from low signal intensity. Metallic nanoparticles such as silver or gold have been used to greatly amplify Raman scattering and have transformed the potential of Raman to be used for applications where sensitivity is critical including detection of diseases based on biomarker identification and identification of trace amounts of illicit substances including drugs and explosives. The future direction of the field is moving towards more in vivo analysis where the unique vibrational signals from SERS offers advantages over complementary techniques such as fluorescence.
Professor Duncan Graham BSc Hons, PhD, CChem, FRSC
FRSE Centre for Molecular Nanometrology Department of Pure and Applied Chemistry Technology and Innovation Centre University of Strathclyde
Enhancement is achieved by adsorbing the sample onto a metal substrate which helps amplify the electric fields of the incident and scattered light. The two main forms of enhancement are chemical and electromagnetic.
Electromagnetic enhancement amplifies the light by exciting the metal’s localised surface plasmon resonances. The metal used traditionally has a roughened surface. This can be achieved using lithography for example. This produces small particles on the nanometre scale which create electromagnetic energy density. This results in an additional electromagnetic field which contributes to the enhancement. The strongest enhancement happens a few nanometres close to the surface and decreases quickly with distance. Nowadays metal coated nano-structure surfaces are commonly used.
Chemical enhancement also helps in some cases but to a lesser extent. Theoretically, chemical enhancement only reaches 103 while electromagnetic enhancement can reach factors as high as 1011.
Chemical enhancement alters the distribution of the charges within the adsorbed molecules which helps create stronger scattering. The enhancement depends on the molecule used so research is aiming to identify those which give a high enhancement.
Optimising Surface-Enhanced Raman Spectroscopy
In carefully optimised systems which combine both types of enhancement, factors of enhancement can reach 1014 . Optimised SERS is powerful enough for single molecule analysis.
Choosing the right metal is key to optimising enhancement. Coinage metals such as silver, gold and copper tend to produce the strongest enhancement. They produce the greatest enhancement in the visible and near-infrared regions which correspond to the regions where Raman spectra are typically observed. Alkali metals are sometimes used but these are very reactive in air unlike silver and gold which are stable.
Using a spectrometer with a laser wavelength that is suitable for the metal is also important. The greatest enhancement tends to occur when the laser wavelength is shifted to the blue of the metal’s plasmon resonance.
The size of the particle being used also impacts their excitation wavelength. For example, silver particles with an average diameter of about 70 nm respond to excitation wavelengths of 488nm.
Reproducing such SERS-active substrates is a key limitation of SERS because typical roughening techniques tend to produce heterogeneous particles. The formation of pinholes in the metal is also a problem. This can be overcome by adsorbing an ultrathin layer of solid or liquid onto the metal and analyte.
In other respects the setup of SERS is the same as normal Raman Spectroscopy’s. It is worth noting that it can produce differences in a material’s spectra compared to normal Raman Spectroscopy.
Applications of Surface-Enhanced Raman Spectroscopy
One of the major advantages of SERS is the fact it can be used to analyse trace amounts of material while still being non-destructive. It also works under ambient conditions, has a broader wavenumber range and can be used in several fields from materials sciences to electrochemistry. Its surface sensitivity means it can used for analysis of biological and organic systems.
It is also useful for a number of real-life applications, such as detecting chemical warfare agents. It has already been used to detect half-mustard gas. It has medical applications such as being used in biosensors to detect biological samples including those involved in cancer and Alzheimer’s disease. It can be used in fraud detection too. For example, identifying dyes at low concentrations in paintings helps date them and therefore authenticate them.
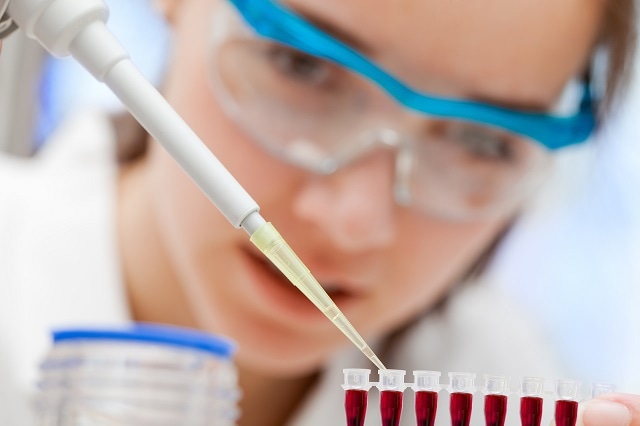
Image Credits: science photo/shutterstock.com
To further increase its uses better instrumentation is key. Portable spectrometers are already available so SERS can be used in the field in forensic science for instance.
Developing new cost effective and reproducible substrates would also increase its use. Aluminium is being investigated as a substrate because its plasmon band is found in the UV region. This could help detect molecules like DNA which respond to ultraviolet light. Novel substrates such as graphene are also being investigated but still present challenges.
Combining SERS with ultrafast spectroscopy techniques to study ultrafast chemical reaction dynamics is also being explored. Tip-enhanced Raman Spectroscopy is another promising area because it can give resolution of features which are only a few nanometres.
Sources and Further Reading
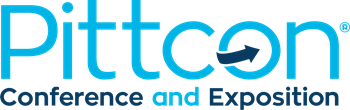
This information has been sourced, reviewed and adapted from materials provided by Pittcon.
For more information on this source, please visit Pittcon.