Engineered nanoparticles (ENP) are said to be widely used in over a thousand products, ranging from cosmetics, clothing, and electronics, to chemical, biomedical, food, energy, materials, environmental, and optical products. The impacts of ENP on human and environmental health are considerably linked to their large surface properties and surface-to-mass ratio.
While the impact of natural colloids on the environment is well researched, there is less understanding about the transport, fate, toxicity and pollutant interactions of ENP. Currently, the tools to analyze these interactions are being designed.
Pollutants-Colloid Interaction
Several nanoparticles suspended in natural water are exposed to proteinaceous materials and pollutants. ENP’s special behaviors and properties are strongly inlfuenced by their physical-chemical properties, including their high surface area relative to their volume, high surface-to-charge ratio density, and high interface energy.
The phase distribution and partitioning of hazardous organic compounds (HOC) can considerably impact the fate and bioavailability of the contaminants in aquatic microorganisms and aquatic systems. There are a broad range of inorganic and organic pollutants that are linked with HOC partitioning to the particles. This partitioning is inversely proportional to the log of particle concentration and log solubility of HOC.
The dynamics of nanoparticle-water partitioning can greatly impact the speciation, and a better insight into the transport, fate, and toxicological effect of POPs - like PCBs and PAHs - is vital. The fate of organic pollutants in aquatic environment often relies on their partitioning behavior to colloids and nanoparticles. Figure 1 shows suspended solid-organic carbon partitioning coefficient versus Kow for polycyclic aromatic hydrocarbons and their derivatives.
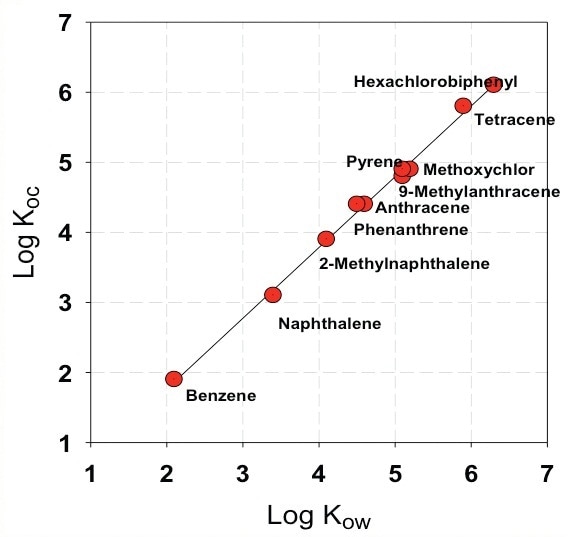
Figure 1. Suspended solid-organic carbon partitioning coefficient versus Kow for polycyclic aromatic hydrocarbons and their derivatives (Karickhoff et. al., Water Res., 241 (1979).
Pollutants Sorbed On Engineered Nanoparticles
The pollutant sorption onto nanoparticles in water phase will be the outcome of three phase partitioning of pollutants between water, organic phase, and suspended solids.
The Octanol-Water Partition Coefficient (Kow): This coefficient refers to the solubility ratio of a compound in octanol (a non-polar solvent) to its solubility in water (a polar solvent). The higher to Kow, the more non-polar the compound. Kd is the ratio of pollutant adhered to the particle and in the water phase.
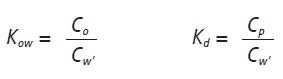
Where Cp, Co, and Cw represent the concentration of the pollutant organic compounds in the non-polar organic phase, in an equal weight of water, and concentration pollutants linked on per mass of particles. Preferably, these ratios are equilibrium partitioning of pollutants between particulate phases, organic phase, and dissolved in water phase.
The physio-chemical properties linked to the environmental behavior of hydrophobic organic compounds are primarily influenced by the octanol-water partition coefficient and aqueous solubility. Aqueous solubility (Sw) represents the equilibrium distribution of a solute between solute and water phases. This means it is the maximum concentration of solute possible at equilibrium, and it can work as a restricting factor in processes that rely on concentration, for instance, kinetic.
The octanol-water partition coefficient is the ratio of a chemical concentration in octanol and in water at equilibrium. Octanol is an organic solvent utilized as a surrogate for organic matter of natural origin. This parameter is applied in several environmental studies to help to establish the outcome of the chemicals in the environment, such as estimating the level at which a pollutant will bioaccumulate in fish.
As the octanol-water partition coefficient is associated with water solubility, a substance’s water solubility can be utilized to predict its octanol-water partition coefficient. The octanol-water partitioning of hydrophobic organic water contaminants can be affected by the presence of nanoparticles.
Measurement Methods
For this research, low molecular weight poly-aromatic hydrocabons (PAHs) was selected including phenanthrene, anthracene, and naphthalene as probe molecules to analyze the transport and outcome of the hydrophobic organic pollutants in water streams nanoparticles (Figure 2).
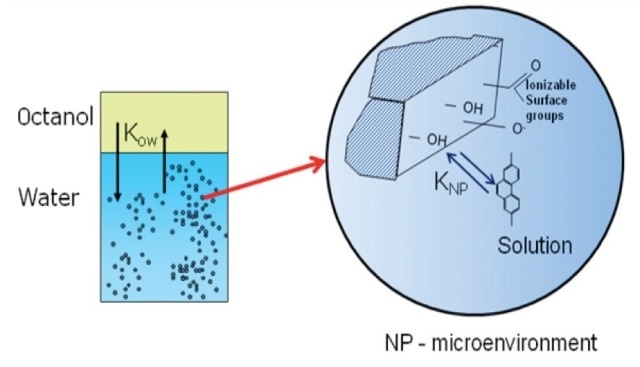
Figure 2. The influence of suspended nanoparticles on octanol-water partitioning of hydrophobic organic pollutants.
In the experimental process, 0-20 mg/L of PAHs in 200 mL of octanol was added to 900 mL of DI water comprising varied concentrations of nanoparticles spanning from 0 to 20 mg/L in an Erlenmeyer flask. The mixture was allowed to settle for 3 hours after stirring the flasks for 5 days and this was followed by separating the octanol and aqueous layers.
The aqueous suspensions were split into three parts. Equal volumes of hexane and methylene chloride (MC) were used to extract one part, followed by centrifuging the mixture and collecting and injecting the supernatant organic phase into the Clarus 600 GC from PerkinElmer (Figure 4).
Gas chromatography analysis was used to measure the PAHs concentrations in water, hexane, and MC. The second part was centrifuged at 10,000 rpm for a period of 30 minutes, the mixture was decanted and the nanoparticles that were settled were placed in a crucible to dry in an oven at 105°C temperature for 8 hours. PerkinElmer’s thermal gravimetric analyzer and TOC or FT-IR were used to examine the mass fraction of the adsorbed organics on dried particles.
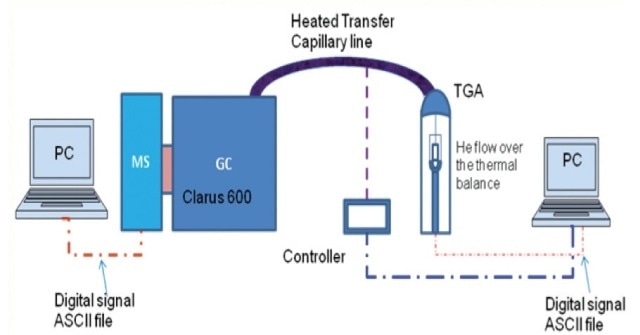
Figure 3. Schematic of PerkinElmer TGA-GC-MS set up with a heated transfer capillary line.
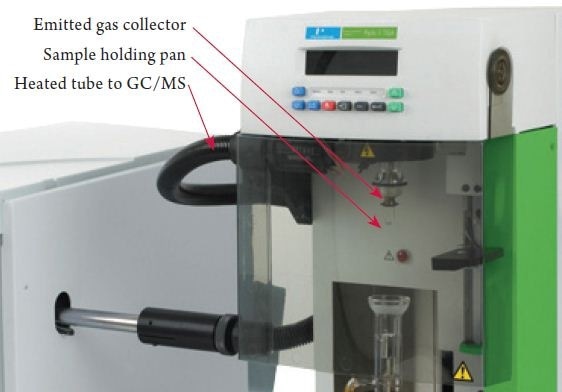
Figure 4. Photograph of PerkinElmer TGA-GC-MS System.
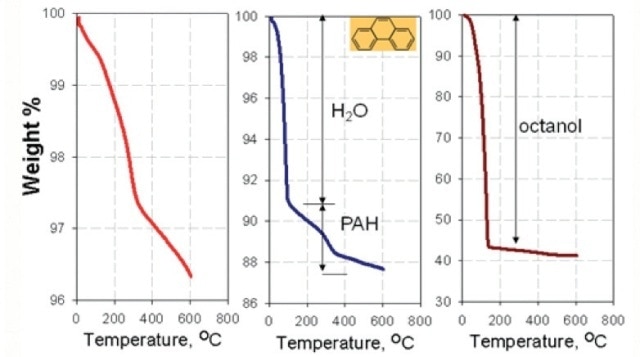
Figure 5. Thermal Analysis of nano-TiTO2, a) conOrganic pollutants adsorbed on nanoparticles: O-W Partitioning.
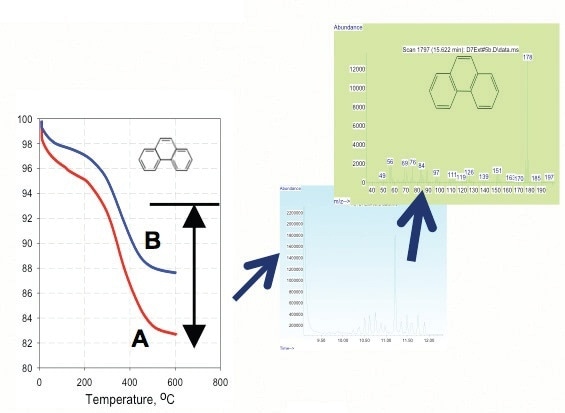
Figure 6. TGA of phenatherene adsorption on nano-TiTO2 at pH of 10 (curve A) and 6.6 (curve B).
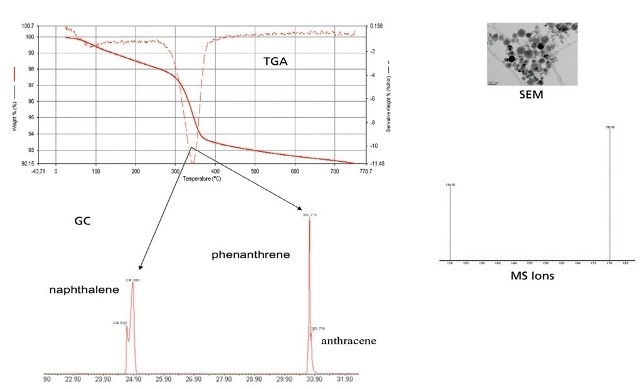
Figure 7. TGA-GC-MS data with SEM image.
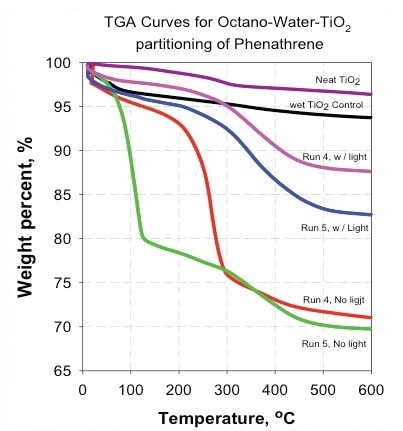
Figure 8. Absence of light made the nanoparticles less hydrophilic and increased the distribution to the organic phase.
Conclusion
- TGA-GC-MS is a practical method to study the effect of nanoparticles on adsorption and partitioning PAHs (Figures 3 and 4).
- The partitioning of PAHs to water phase is increased by the presence of nano-TiO2.
- Nanoparticles lead to increased partitioning of PAHs that is 6 to 10 times more than in water-octanol equilibrium conditions (Figure 5).
- Phenanthrene and naphthalene exhibit low water solubility yet differing partitioning (Figure 6).
- Partitioning effect increases at higher pH (Figure 7).
- The absence of light makes the nanoparticles less hydrophilic and increases the distribution to the organic phase (Figure 8).
- The study suggests that the environmental risk of NP should include their transformation and influence in the transport of other pollutants.
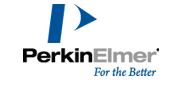
This information has been sourced, reviewed and adapted from materials provided by PerkinElmer Inc.
For more information on this source, please visit PerkinElmer Inc.