The increased use of nanoparticles (NPs) in consumer products and industrial processes has also increased the chances that the NPs will enter the environment from discarded consumer products and via the release of industrial waste.
Even though their concentration is anticipated to be very low in the environmental systems, it is not known if they affect human health. So there is a need to determine the presence on NPs in drinking water systems.
It's unique ability to perform sensitive, fast, and element-specific analysis makes the Single Particle ICP-MS, (SP-ICP-MS) a perfect tool for the measurement of NPs in drinking water systems. This article discusses the efficiency of drinking water treatment systems to eliminate silver (Ag), gold (Au), and titanium dioxide (TiO2) NPs by employing SP-ICP-MS as the only analytical method.
Experimental Procedure
Chemicals and Materials
Au and Ag NPs were purchased from nanoComposix™ (San Diego, CA, USA) and TiO2 NPs were obtained from US Research Nanomaterials, Inc. (Houston, TX, USA). The size and characteristics of the NPs used in this analysis are listed in Table 1. Intermediate suspensions were formed in ultra-high purity water to disperse any agglomerated particle clusters.
Table 1. Nanoparticle characteristics
Material |
Diameters (nm) |
Characteristics |
Gold (Au) |
50, 80, 100 |
Capped with citrate |
Silver (Ag) |
40, 70, 100 |
Capped with citrate |
Titanium dioxide (TiO2) |
100, 160 |
Uncapped |
Water samples, such as ultra-pure water, source water (surface water from Missouri River), and drinking water were taken for the analysis post-treatment from three water treatment plants.
Instrumental Conditions
Each analysis was carried out using a PerkinElmer NexION 300D/350D ICP-MS, including Syngistix software and the Syngistix Nano Application Module. The instrumental and method parameters used for analysis are listed in Table 2. Gravimetric measurement of the sample uptake rate was carried out, and the Au NPs were taken as the reference to determine transport efficiency.
Table 2. Instrument and method parameters for single particle ICP-MS analysis of Au, Ag, and TiO2 in single particle mode
Instrument Parameter |
Operation Setting |
Nebulizer |
Concentric |
Spray Chamber |
Cyclonic |
Cones |
Platinum |
RF Power (W) |
1400 |
Nebulizer Gas Flow (L/min) |
1.02-1.06 |
RPq |
0.5 |
Sample Uptake Rate (mL/min) |
0.26-0.29 |
Dwell Time (µs) |
100 |
Sample Time (s) |
100 |
Transport Efficiency (%) |
7.5-8.5 |
Method Parameters |
Au |
Ag |
Ti |
Isotope (amu) |
196.967 |
106.905 |
46.9518 |
Density (g/cm3) |
19.3 |
10.49 |
4.23 |
Mass Fraction (%) |
100 |
100 |
60 |
Ionization Efficiency (%) |
100 |
100 |
100 |
All of the measurements were performed against external calibration curves generated by the Syngistix Nano Application Module. Calibration standards for the dissolved concentrations were prepared by carrying out serial dilutions of single-element stock solutions. Particle calibration standards were prepared by diluting the suitable particles to concentrations less than or equal to roughly 105 particles/mL in order to reduce the possibility of multiple particles reaching the plasma at the same time.
Results and Discussion
Detection Limits
Table 3. illustrates the dissolved and particle size detection limits in surface water, they were determined by preparing the NP suspension in filtered river water through the SP-ICP-MS mode. The dissolved detection limits are greater than the limits acquired through conventional ICP-MS measurements due to the shorter integration times employed: 100 µs in this study vs typically 1 second or greater for conventional ICP-MS measurements.
The particle size detection limit for TiO2 is higher than that for Ag and Au because of the particle composition: Ag and Au particles are pure metal, but TiO2 particles contain only 60% Ti. Despite of the mere 7.4% abundance, 47Ti+ was selected to make the titanium measurements instead of the most abundant titanium isotope (48Ti+ with 73.8% abundance) because Ca has an isotope at m/z 48 as well. As increased levels of Ca are also found in drinking water, it might interfere with Ti at this mass.
Table 3. Detection Limits
Element |
Dissolved (µg/L) |
Particle (nm) |
Ag |
0.10 |
23-25 |
Au |
0.10 |
28-30 |
TiO2 |
0.75 |
65-70 |
To analyze the effectiveness of the water treatment procedure employed in the treatment plants to eliminate Ag, Au, and TiO2 NPs, water samples both pre- and post-treatment were collected at three water treatment plants. Not even one out of the six water samples contained assessable amounts of Ag or Au, either dissolved or as particles.
As illustrated in Table 4, all of the samples consisted of TiO2. Plants 1 and 2 eliminated dissolved Ti and TiO2 particles efficiently, as indicated by the lower amounts in the post-treatment than the pre-treatment waters. Although considerably less present in pre-treatment, Ti-containing particles could be detected even after treatment from the Plant 3 results, but all the dissolved Ti was eliminated.
Table 4. Effectiveness of three water treatment plants removing TiO2 particles and dissolved Ti
Plant |
Pre/Post Treatment |
Most Frequent Size (nm) |
Particle Concentration (particles/mL) |
Dissolved Concentration (µg/L) |
1 |
Pre
Post |
170
< MDL |
432,000
< MDL |
17.9
1.21 |
2 |
Pre
Post |
156
< MDL |
451,000
< MDL |
11.7
1.17 |
3 |
Pre
Post |
153
76 |
425,000
17,237 |
10.6
< MDL |
To examine the accuracy, spike recovery tests for all dissolved and NP metals were carried out. Three samples were spiked by using 100 nm NPs and 2 µg/L of the dissolved metals at a concentration of ≈1 x 105 particles/mL. The results of the spike recovery tests are illustrated in Table 5 and denote accurate recoveries, but show low recovery for TiO2 NPs concentration, which recovered less than 10% for each of the samples. This was because of the aggregation in the standards and in the water matrix.
In the absence of a surfactant, the uncapped TiO2 particles tended to aggregate, resulting in particle loss between dilutions and in the water matrix, where they had the scope to react and form new species, and/or aggregating further and falling out of the solution. The highest recovery percentages for these particles in water matrix and in ultra-pure water were 9% and 24%, respectively.
Table 5. Spike recovery studies in drinking waters.
Sample |
Au |
Ag |
TiO2 |
Most Freq Size (nm) |
Part Conc. Spike Recovery |
Diss Conc. Spike Recovery |
Most Freq Size (nm) |
Part Conc. Spike Recovery |
Diss Conc. Spike Recovery |
Most Freq Size (nm) |
Part Conc. Spike Recovery |
Diss Conc. Spike Recovery |
1 |
98 |
97% |
80% |
98 |
97% |
80% |
102 |
9% |
84% |
2 |
97 |
88% |
84% |
97 |
88% |
84% |
87 |
6% |
88% |
3 |
101 |
94% |
89% |
101 |
94% |
89% |
87 |
6% |
112% |
Conclusion
This article has clearly showed the ability of the NexION 350 ICP-MS operating in Single Particle mode to precisely detect Ag, Au, and TiO2 NPs in drinking water systems. The analysis of real samples, both pre- and post-treatment, indicated that drinking water treatment procedures of the examined water treatment plants effectively eliminated both Ti-containing and dissolved NPs. However, neither Ag nor Au was detected in these samples.
The application of the Syngistix Nano Application Module along with the NexION 350 working in Single Particle mode is a perfect tool for the continuous and rapid analysis of NPs in real samples, specifically for analyzing samples with lower particle concentrations.
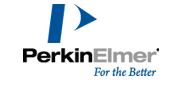
This information has been sourced, reviewed and adapted from materials provided by PerkinElmer Inc.
For more information on this source, please visit PerkinElmer Inc.