Luminescent metal-organic frameworks (LMOFs) are a class of MOF materials that have received much research interest across various fields. However, conventional techniques employed to produce them find it difficult to overcome challenges associated hybrid-organic nature. Research published in the journal Optics Express puts forth a new femtosecond laser based technique to synthesize LMOFs.
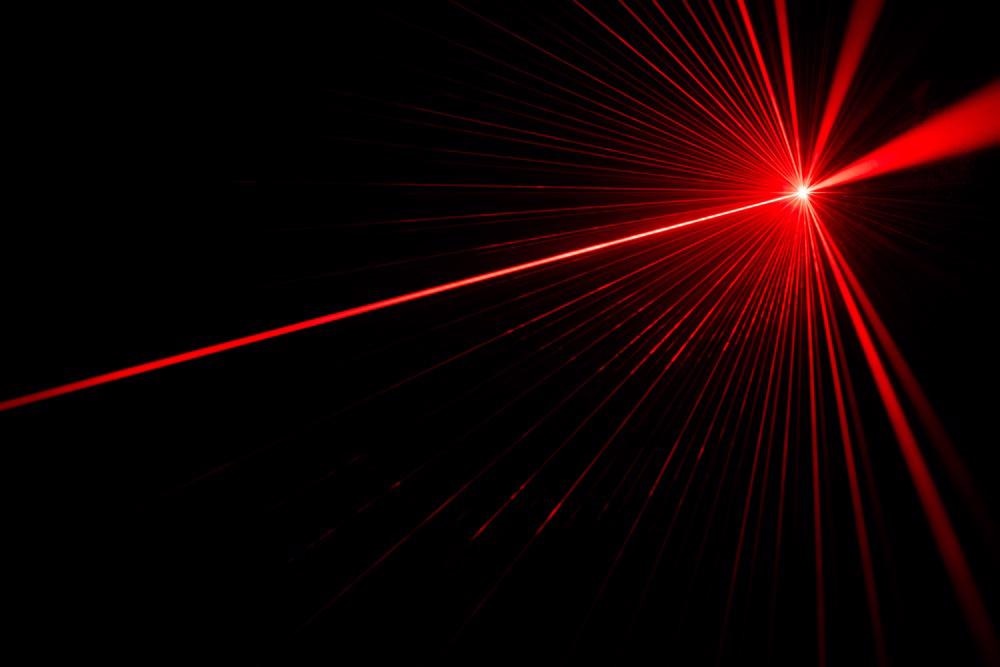
Image Credit: donatas1205/Shutterstock.com
MOFs are compounds containing metal ions coordinated to organic ligands, have well-controlled porosity, high surface area, and electrochemical properties. Their use covers various fields like biomedical sensing, light-emitting techologies, and catalysts.
MOFs are widely synthesized through solvothermal synthesis, traditionally done in a Teflon reactor with a convention oven technique. However, this technique is time-consuming.
These methods produce crystallized powders, which can be integrated into devices via patterning techniques; micro-contact printing, inkjet printing, and photolithography are typical examples. Patterning approaches are a complicated, multistep process, taking place under harsh conditions. Because of this, performance degradation of the final product is often observed.
Here, researchers detail the usefulness of femtosecond laser-induced in-situ crystallization of Terbium (Tb)-based luminescent metal-organic framework, introducing this method into directly patterning MOFs for the first time.
The potential for femtosecond laser techniques in delivering the fast fabrication and integration of MOFs is evidenced.
Methodology
The Tb(BTC)·G (BTC = 1,3,5-Benzenetricarboxylic acid and G = guest solvent) was generated by dissolving metal salt and an organic ligand into an appropriate solvent to form a precursor solution which was irradiated by multiple femtosecond laser pulses.
The technique puts chemical synthesis and device integration into a single step, reducing the creation time from hours to seconds or even milliseconds.
Chemicals like Terbium(III) nitrate hexahydrate, N, N-dimethylformamide, 1,3,5-Benzenetricarboxylic acid, N-Methyl pyrrolidone were mixed and heated to 60 °C under vigorous stirring for 5 minutes to prepare the precursor sample. The resultant mixture was filtered using a polytetrafluoroethylene membrane filter.
The experiments took place on a homemade direct writing system with a femtosecond laser (532nm wavelength, pulse duration 170fs).
Tb(BTC)·G micro-disks were produced by moving the sample using a piezo XYZ stage while maintaining a stable laser beam.
Morphological characterization of the Tb(BTC)·G micro-disk arrays was carried out with a high-resolution field-emission scanning electron microscope. The high-resolution transmission electron microscopy (HRTEM) images, selected area electron diffraction (SAED), energy dispersive spectrum (EDS), patterns, and elemental mapping analysis were also conducted and noted by a Titan G2 60-300 transmission electron microscope.
The photoluminescence images were obtained with the help of a homemade wide-field fluorescence microscope. An ultraviolet-visible-near infrared spectrophotometer was used to register the absorption spectra of the NMP solution.
Results
In this study, a femtosecond laser was employed as the energy source to cause a highly confined photon synthesis reaction region, which triggered the in-situ crystallization of the MOF samples. Figure 1 illustrates the femtosecond in-situ laser-induced crystallization of Tb(BTC)·G.
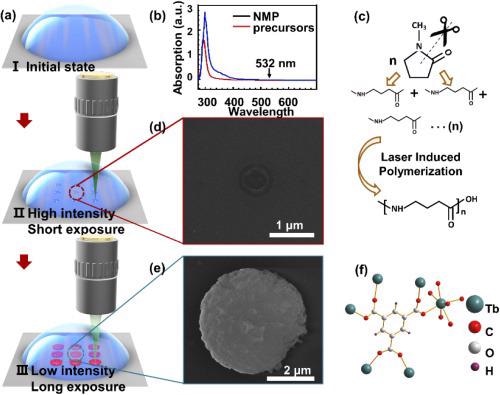
Figure 1. Schematic of the femtosecond laser-induced in-situ crystallization of Tb(BTC)·G. (a) schematic of the laser writing process: I. the initial state of the precursor, II. high intensity and short exposure laser-induced dot arrays of polymerized NMP, III. low intensity and long exposure laser-induced dot arrays of Tb(BTC)·G; (b) absorption curve of the NMP solvent and the precursor; (c) chemical reaction of the NMP under high intense irradiation of the femtosecond laser; (d) SEM image of polymerized NMP; (e) SEM image of Tb(BTC)·G; (f) the molecular structure of the Tb(BTC)·G. Image Credit: Liu, et al., 2021
Enabling in-situ crystallization of Tb(BTC)·G with the femtosecond laser requires a suitable solvent; it must be optically transparent to the exciting wavelength and dissolve the organic ligand and metal salt; possess a high boiling point.
The most widely used solvents are ethanol, water, N-methyl pyrrolidone (NMP), and N, N-dimethylformamide (DMF).
Various Tb(BTC)·G microdisk arrays were produced on a glass substrate by the proposed technique (see Figure 2).
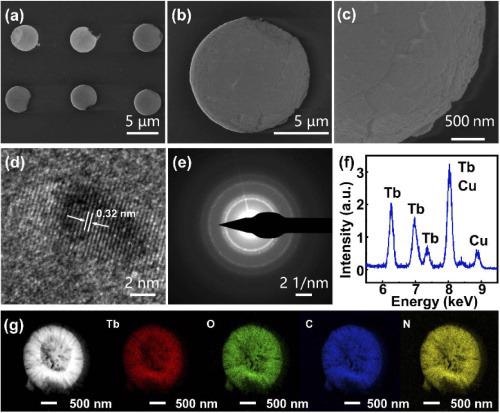
Figure 2. (a, b, c) SEM images of the Tb(BTC)·G dot arrays, (d) HRTEM of Tb(BTC)·G, (e) SAED patterns of Tb(BTC)·G, (f) EDS of Tb(BTC)·G, (g) elemental mapping analysis of Tb(BTC)·G. Image Credit: Liu, et al., 2021
Various sizes of micro Tb(BTC)·G structures were produced by tuning the laser power and the exposure time. The influence of the laser exposure time on the size of Tb(BTC)·G was later analyzed. Figure 3a illustrates the SEM image of the Tb(BTC)·G dot.
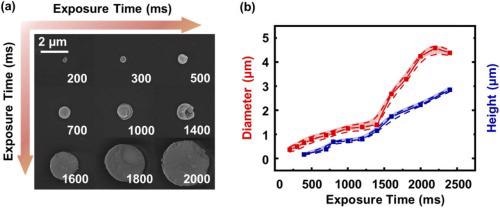
Figure 3. (a) SEM image of Tb(BTC)·G dot arrays with different exposure times. (b) the diameter and height of Tb(BTC)·G change with different exposure times. Image Credit: Liu, et al., 2021
Synthesis of metal organic frameworks can range between minutes to several days when traditional methods are used. In this research, a new approach utilizing femtosecond laser methods is proposed, which demonstrates a significantly reduced processing time.
This reduced timeframe is thought to be attributed to the laser's fast energy injection, which is then absorbed by the precursor where it is converted to heat energy.
In rare-earth MOFs, fluorescence is an attractive character. Rare earth MOFs combine rare earth metal ions and organic ligands which facilitates a broad range of photon emission phenomena like metal-based emission, linker-based luminescence, and other more complicated emissions.
The produced Tb(BTC)·G with a femtosecond laser shows excellent fluorescence properties (see Figure 4).
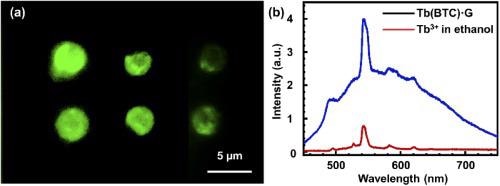
Figure 4. (a) fluorescence image of the Tb(BTC)·G, (b) fluorescence spectrum of Tb(BTC)·G (red) and Tb ions in ethanol. Image Credit: Liu, et al., 2021
Conclusion
The study demonstrated a femtosecond laser triggered in-situ crystallization of Tb-based metal-organic frameworks. Samples were produced at target positions within milliseconds, a significant improvement to conventional solvothermal processes.
Researchers also found that by varying variables such as exposure time, the size of the Tb(BTC)·G samples could be changed.
The strong fluorescence of the prepared Tb(BTC)·G indicates their potential in a range of optoelectronic applications, though future work will be required to deconstruct this fluorescence mechanism.
The proposed approach offers a means to synthesize and integrate MOFs in a single step, enhancing the creation of miniature devices based on MOF materials.
Continue reading: A Guide to AFM Nanotips.
Journal Reference
Liu, Y., Chai, N., Wang, X., Luo, Z., Zhao, J., Xie, C., Gan, Z. (2021) Femtosecond laser induced in-situ crystallization of Tb-based luminescent metal organic framework. Optics Express, 29(24), pp. 39304–39311. Available online: https://doi.org/10.1364/OE.411315.
References and Further Reading
- Chae, H. K., et al. (2004) A route to high surface area, porosity and inclusion of large molecules in crystals. Nature, 427(6974), pp. 523–527. doi.org/10.1038/nature02311.
- Liu, T.-F., et al. (2015) Topology-guided design and syntheses of highly stable mesoporous porphyrinic zirconium metal–organic frameworks with high surface area. Journal of American Chemical Society, 137(1), pp. 413–419. doi.org/10.1021/ja5111317.
- Honicke, I.-M., et al. (2018) Balancing Mechanical Stability and Ultrahigh Porosity in Crystalline Framework Materials. Angewandte Chemie, 57(42), pp. 13780–13783. doi.org/10.1002/anie.201808240.
- Furukawa, H., et al. (2010) Ultrahigh porosity in metal-organic frameworks. Science, 329(5990), pp. 424–428. doi.org/10.1126/science.1192160.
- Liao, P.-Q., et al. (2018) Metal-organic frameworks for electrocatalysis. Coordination Chemistry Reviews, 373, pp. 22–48. doi.org/10.1016/j.ccr.2017.09.001.
- Cai, Z.-X., et al. (2019) Hollow Functional Materials Derived from Metal-Organic Frameworks: Synthetic Strategies, Conversion Mechanisms, and Electrochemical Applications. Advanced Materials, 31, p. 1804903. doi.org/10.1002/adma.201804903.
- Sava, D.-F., et al. (2012) Intrinsic Broad-Band White-Light Emission by a Tuned, Corrugated Metal-Organic Framework. Journal of American Chemical Society, 134(9), pp. 3983–3986. doi.org/10.1021/ja211230p.
- Sun, C.-Y., et al. (2013) Efficient and tunable white-light emission of metal–organic frameworks by iridium-complex encapsulation. Nature Communications, 4(1), p. 2717. doi.org/10.1038/ncomms3717.
- Kreno, L.-E., et al. (2012) Metal–organic framework materials as chemical sensors. Chemical Reviews, 112(2), pp. 1105–1125. doi.org/10.1021/cr200324t.
- Meng, J., et al. (2020) Advances in metal-organic framework coatings: versatile synthesis and broad applications. Chemical Society Reviews, 49(10), pp. 3142–3186. doi.org/10.1039/C9CS00806C.
- Rosi, N.-L., et al. (2005) Rod packings and metal-organic frameworks constructed from rod-shaped secondary building units. Journal of American Chemical Society. 127(5), pp. 1504–1518. doi.org/10.1021/ja045123o.
- Khan, N.-A., et al. (2015) Synthesis of metal-organic frameworks (MOFs) with microwave or ultrasound: Rapid reaction, phase-selectivity, and size reduction Coordination Chemistry Reviews, 285, pp. 11–23. doi.org/10.1016/j.ccr.2014.10.008.
- Da Luz, L. L., et al. (2015) Inkjet printing of lanthanide–organic frameworks for anti-counterfeiting applications. ACS Applied Materials Interfaces, 7(49), pp. 27115–27123. doi.org/10.1021/acsami.5b06301.
- Arslan, H.-K., et al. (2011) High-Throughput Fabrication of Uniform and Homogenous MOF Coatings. Advanced Functional Materials, 21(22), pp. 4228–4231. doi.org/10.1002/adfm.201101592.
- Lu, G., et al. (2012) Engineering ZIF-8 Thin Films for Hybrid MOF-Based Devices. Advanced Materials, 24(29), pp. 3970–3974. doi.org/10.1002/adma.201202116.
- Zhuang, J., et al. (2012) The oriented and patterned growth of fluorescent metal–organic frameworks onto functionalized surfaces. Beilstein Journal of Nanotechnology, 3, pp. 570–578. doi.org/10.3762/bjnano.3.66.
- Thakkar, H., et al. (2017) 3D-Printed Metal-Organic Framework Monoliths for Gas Adsorption Processes. ACS Applied Materials Interfaces, 9(41), pp. 35908–35916. doi.org/10.1021/acsami.7b11626.
- Huangfu, M., et al. (2019) Post-synthetic modification of a Tb-based metal-organic framework for highly selective and sensitive detection of metal ions in aqueous solution. New Journal of Chemistry, 43(26), pp. 10232–10236. doi.org/10.1039/C9NJ01835B.
- Li, B., et al. (2016) Emerging Multifunctional Metal-Organic Framework Materials,” Advanced Materials, 28(40), pp. 8819–8860. doi.org/10.1002/adma.201601133.
- Yang, Q., et al. (2017) Metal-organic frameworks meet metal nanoparticles: synergistic effect for enhanced catalysis. Chemical Society Reviews, 46(15), pp. 4774–4808. doi.org/10.1039/C6CS00724D.
- Von der Linde, D., et al. (1997) Laser-solid interaction in the femtosecond time regime. Applied Surface Science, 109–110, pp. 1–10. doi.org/10.1016/S0169-4332(96)00611-3.
- Eaton, S. M., et al. (2005) Heat accumulation effects in femtosecond laser-written waveguides with variable repetition rate. Optics Express, 13(12), pp. 4708–4716. doi.org/10.1364/OPEX.13.004708.
- Liu, Y., et al. (2019) General and Fast Patterning of Semiconductor Nanocrystals by Femtosecond Laser Direct Writing. Journal of Physics D: Applied Physics. doi.org/10.1088/1361-6463/ab6623.
- Liu, Y., et al. (2019) Fluorescent Microarrays of in Situ Crystallized Perovskite Nanocomposites Fabricated for Patterned Applications by Using Inkjet Printing. ACS Nano, 13, pp. 2042–2049. doi.org/10.1021/acsnano.8b08582.
- Cui, Y., et al. (2009) Luminescent metal-organic frameworks. Chemical Society Reviews, 38(5), pp. 1330–1352. doi.org/10.1039/B802352M.
- Cui, Y., et al. (2012) Luminescent functional metal-organic frameworks. Chemical Reviews, 112(2), pp. 1126–1162. doi.org/10.1021/cr200101d.
- Yin, H.-Q., et al. (2019) Rotation Restricted Emission and Antenna Effect in Single Metal-Organic Frameworks. Journal of American Chemical Society, 141(38), pp. 15166–15173. doi.org/10.1021/jacs.9b06755.