Gas sensor technology is employed in many applications, including home security, air pollution control, health monitoring, food safety, and industrial automation. Advances in the development of nanostructured thin films have enabled the production of gas nanosensors with better sensitivity, selectivity, and faster response times at a lower cost.
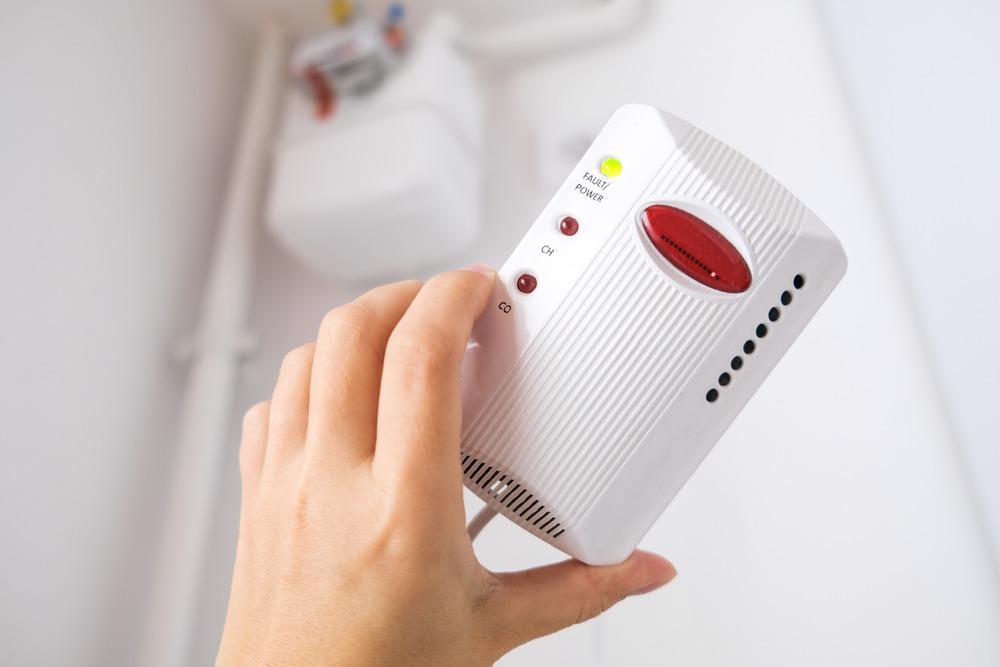
Image Credit: juliet_dreamhunter/Shutterstock.com
Gas sensors are critical for monitoring and detecting hazardous and inert gases or analyzing the environment in many different industrial and consumer applications. Gas detection and monitoring have always been closely connected with protecting working environments where the risk of exposure to dangerous gases is high.
Past, Current, and Future Use of Gas Sensors
Historically, gas sensors were first used in coal mines where accurate monitoring of hazardous gases must be carried out continually. Soon gas sensors were also beginning to appear in the chemical industry and environmental pollution monitoring.
Gas sensors are widely used in the healthcare industry for accurate, reliable, and consistent monitoring of anesthetic and respiratory gases during operations. Another widespread application of gas sensors is air quality monitoring.
The devices are used to monitor the levels of CO, CO2, and other harmful gases in homes, office buildings, classrooms, vehicles, and enclosed environments.
Commercial greenhouse agriculture relies on gas sensing technology to control CO2 concentration for optimal crop growth. New emerging applications, such as modified atmosphere packaging and controlled atmosphere storage that enable fresh and minimally-processed foods to maintain visual, textual, and nutritional appeal, require constant monitoring of oxygen, CO2, and nitrogen concentrations during packaging and storage.
Advances in Materials Research Help to Meet Challenges
With the ever-expanding range of demanding applications, modern gas sensors have imposed more challenging specifications.
The most widely-used sensing materials have evolved from metal oxides, which still play a dominant role in modern gas sensors.
Metal oxide gas sensors are chemiresistive devices where the resistance of the material changes as a result of exposure to the target gas. The change in the resistance depends on the interaction of conduction band electrons of the material with oxygen ions.
Such a sensing mechanism permits the metal oxide gas sensors to detect oxidizing and reducing gases in the environment.
The main drawbacks of the metal oxide gas sensors are the cross-sensitivity (detecting several redox reactions) and the high operating temperature needed to overcome the activation barrier of the sensing material, which greatly improves the sensing performance of the material.
Gas sensors with lower sensitivity are disadvantageous for real-time applications, while higher operating temperatures increase the power consumption of the device.
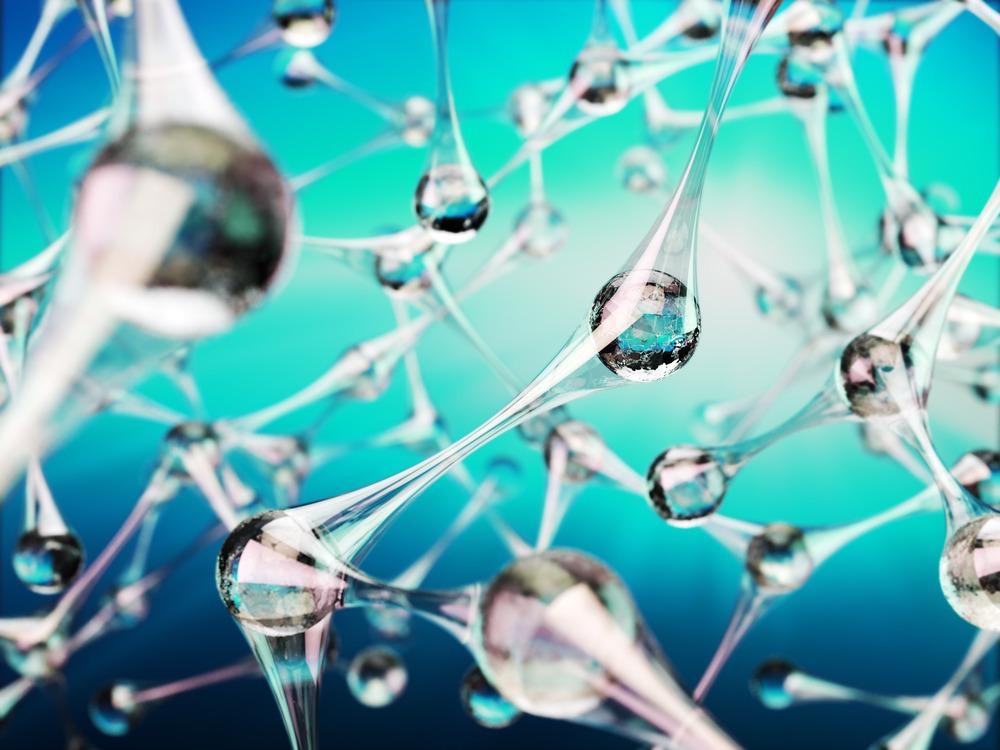
Image Credit: cybrain/Shutterstock.com
How Nanostructured Materials Can Improve Gas Sensor Performance?
The rapid development of nanotechnology in the past two decades demonstrated that nanostructured materials can greatly improve the performance of gas sensors owing to their extremely high surface-to-volume ratio and high charge carrier mobility.
The large surface area to volume ratio of the nanostructured materials increases the probability for these surface reactions to occur. This, in turn, increases the sensitivity of the gas sensor while reducing its response time.
The synthesis of nanostructured metal oxides has recently attracted a lot of interest as various top-down and bottom-up nanofabrication methods, such as hydrothermal synthesis, electrochemical deposition, magnetron sputtering deposition, and chemical vapor deposition, enable scientists to create a variety of metal oxide morphologies.
This includes nanoparticles, nanowires, nanorods, nanocrystals, nanostructured thin films, and others.
The morphology of these solid-state metal oxide nanostructures and the high charge mobility on their surface makes them extremely sensitive to the composition of the surrounding atmosphere.
Nanosensors Based on Nanostructured Metal Oxide Materials
For example, the nanostructured morphology of lanthanum oxide (Ln2O3) plays a significant role in determining its electrochemical and optical properties.
Ln2O3-based nanosensors with different morphologies demonstrated accurate and selective sensing of harmful or toxic volatile compounds produced from the chemical industry, including formaldehyde, acetone, toluene, H2S, NH3, CO, O3, Cl2, H2, CO, and NO2.
Nanostructured titanium oxide (TiO2) is another promising material for gas-sensing applications owing to its optical and electronic properties, resulting in low-temperature sensitivity towards H2 and CO.
The use of n-type semiconductor nanomaterials, such as tin dioxide (SnO2) and zinc oxide (ZnO), permits the detection of changes in the material's resistance resulting from adsorbed surface species.
Such a sensing mechanism, based on the amount of absorbed oxygen, enables the detection of various types of toxic gases and organic vapors.
The sensitivity and selectivity of the nanostructured metal oxides can be tuned by adding dopants or noble metal catalysts and reaction promoters (Pd, Pt, Ag, Au, and others).
The catalytic action of metal nanoparticles results in the dissociation of molecular oxygen into ionic oxygen species that get adsorbed on the surface of the nanosensor and react with the targeted gas molecules.
Indium and ruthenium dopants are widely used in SnO2-based nanosensors to improve sensing toward NO2 and lower the sensor's operating temperature.
A Promising Future for Carbon Nanomaterial Sensors
In recent years, researchers started looking for alternatives to conventional metal oxide sensing materials. The use of carbon nanomaterials offers promising results in the field.
Owing to their low cost, ease of processing, high mechanical strength, and good thermal stability, materials like graphene and its derivatives (graphene oxide and reduced graphene oxide) are being used increasingly in gas sensing applications.
The interaction between the graphene sheets and the target gas molecules varies from weak van der Waals interactions to strong covalent bonding.
Their excellent ballistic conductivity, high charge carrier mobility at room temperature, and low electrical noise due to graphene derivatives' unique two-dimensional honeycomb structure enable facile monitoring of the above-mentioned interactions by simple and convenient electronic methods.
Recent studies have shown that the graphene materials exhibit different changes in their resistivity depending on the type of the adsorbed gases, where the sign of the change indicated whether the gas was an electron acceptor like NO2, H2O, or an electron donor like NH3, CO, and ethanol.
Unlike the existing sensors, the carbon-based nanosensors can offer a highly effective multi-species gas sensing with a faster response time at a lower cost and reduced power consumption.
Continue reading: Nanostructured Thin Films in Gas Sensor Devices
References and Further Reading
Chen, X., et al. (2021). Nanostructured Gas Sensors: From Air Quality and Environmental Monitoring to Healthcare and Medical Applications. Nanomaterials (Basel, Switzerland), 11(8), 1927. Available at: https://doi.org/10.3390/nano11081927
Javaid, M., et al. (2021). Exploring the potential of nanosensors: A brief overview. Sensors International, 2, 100130. Available at: https://doi.org/10.1016/j.sintl.2021.100130
Chowdhury, N. K. and Bhowmik, B. (2021) Micro/nanostructured gas sensors: the physics behind the nanostructure growth, sensing and selectivity mechanisms. Nanoscale Adv., 3, 73-93. Available at: https://doi.org/10.1039/D0NA00552E
Wang, H., et al. (2021) Gas sensing materials roadmap. J. Phys.: Condens. Matter, 33, 303001. Available at: https://doi.org/10.1088/1361-648x/abf477
Casanova-Cháfer, J., et al. (2019) Gas Sensing Properties of Perovskite Decorated Graphene at Room Temperature. Sensors, 19, 4563. Available at: https://doi.org/10.3390/s19204563
Disclaimer: The views expressed here are those of the author expressed in their private capacity and do not necessarily represent the views of AZoM.com Limited T/A AZoNetwork the owner and operator of this website. This disclaimer forms part of the Terms and conditions of use of this website.