Sponsored by MerckReviewed by Emily MageeJan 23 2024
Colloidal quantum dots (CQDs) are semiconducting crystals. They exist on a nanometer scale (ca. 2–12 nm) and are coated with ligand/surfactant molecules to avoid agglomeration.
Due to their size, CQDs can provide unique properties through quantum confinement.1 By adjusting the size, shape, and composition of a QD, it is possible to attain highly tunable optical and electrical properties, enabling the utilization of QDs in a wide range of applications.
QDs are particularly promising in optoelectronic devices such as photovoltaics, light-emitting diodes, lasers, photodetectors, and field-effect transistors.2–4
Since researchers initially described the size-dependent properties of QDs in the early 1980s, many different QD nanocrystals have surfaced.5,6 Substantial advancements have been made in semiconductor particles, particularly concerning colloidal synthesis methods.
The advancement of CQDs has been especially promising in solution-processable optoelectronic devices. For instance, those involved in ink-jet printing, spin coating, screen printing, and blade coating have paved the way for the affordable fabrication of large-area flexible devices.7
However, these advancements have not been devoid of challenges. Over the last three decades, developments in CQD production methodologies have led to the evolution of various methods to synthesize them with uniform size.
Monodisperse CQDs are significant as they have homogeneous properties that can be employed in high-quality optoelectronic devices.
This article will summarize several methods to synthesize CQDs, focusing on the evolution of monodisperse cadmium- and lead-based CQDs—specifically CdSe, CdTe, PbS, and perovskite QDs—due to their widespread use and effective properties.
Numerous methods for the batch synthesis of monodisperse QDs—including hot-injection, heat-up, cluster-assisted, and microwave-assisted synthesis approaches—will be outlined below.
This article also evaluates the use of continuous flow-assisted synthesis for large-scale production of CQDs, comparing its advantages with those of the batch approach.
The Hot-Injection Method
The methods currently used to synthesize monodisperse CQDs are based predominantly on the work of LaMer and Dinegar, who described how rapid nucleation and regulated growth of the existing nuclei determine the production of monodisperse colloids.8,9
Utilizing this approach, Alivisatos and Bawendi et al. established the “hot-injection” method of synthesizing monodisperse CdS, CdSe, and CdTe QDs.10,11
The hot-injection approach continues to be the most common method for synthesizing monodisperse nanocrystals. It involves the rapid injection of organometallic reagents into a hot solvent, generating homogeneous nuclei.
The reaction solution utilized during this process contains surfactant molecules/ligands to stop QDs from agglomerating. The ligands that are typically used include alkylphosphine and alkylphosphine oxides (such as trioctylphosphine, Prod. No. 718165 and trioctylphosphine oxide, Prod. No. 223301), long-chain amines (such as oleylamine), and long-chain carboxylic acids (such as oleic acid, Prod. No. O1008).2
After nucleation, a homogeneous diffusion-controlled growth is evident across the solution, during which the smaller QDs grow quicker than the larger QDs, leading to a size-focusing effect.
Ostwald ripening occurs as the growth continues—that is, the larger QDs continue to grow while the smaller QDs dissolve because of their higher chemical potential.4 The particle concentration decreases and the average particle size increases until the point of saturation.
The hot-injection method provides a high level of control over the size of the particles and size distribution by enabling a rapid nucleation separation from the growth stage. It is therefore a particularly effective method.
By adjusting the concentration of the surfactants, temperature, and reaction time, it is possible to synthesize QDs of various sizes. This method has also proven effective at synthesizing different QD nanocrystal types.
The hot-injection approach has recently been adapted to synthesize all-inorganic cesium lead halide perovskite (CsPbX3, X = Cl, Br, I) nanocrystals.12
CsPbX3 nanocrystals can be synthesized by reacting Cs-oleate with a Pb(II)-halide in octadecene (Prod. No. 74740) under N2 atmosphere at a high temperature (between 140 °C and 200 °C).
Before the Cs-oleate is injected, oleic acid and oleylamine (mixed at a 1:1 ratio) are added to the Pb(II)-halide solution in octadecene at N2 atmosphere and 120 °C. This solubilizes the Pb(II)-halide and stabilizes the obtained nanocrystals.
Due to the nature of the ionic metathesis reaction in CsPbX3nanocrystals, the growth kinetics and nucleation are extremely quick—growth occurs within 1–3 seconds.
The size of the CsPbX3 nanocrystals can therefore be tuned easily by changing the reaction temperature, but not by changing the reaction time. The CsPbX3 nanocrystals synthesized via this method display bandgap energies tunable by composition and size.
This approach can be used to synthesize CsPbX3 nanocrystals that cover the whole visible spectral region with narrow emission line widths (12–42 nm) and display a photoluminescence quantum yield (PLQY) ca. 50–90%.
The Heat-Up Method
The heat-up method involves gradually heating the precursors in the presence of a ligand. It is a non-injection approach and is largely considered to be more efficient at preparing nanocrystals than other methods, as it enables them to be prepared in an individual pot without an injection stage.
Most heat-up synthesis methods yield a polydisperse particle size distribution due to the spread of nucleation events over a considerable period. Cao et al. reported that this method could be utilized to obtain monodisperse CQDs if precursors with an appropriate reactivity level are used.
Similar to the hot-injection method, the essential step is to utilize a homogeneous nucleation process by using highly reactive precursors only when they reach a specific temperature.
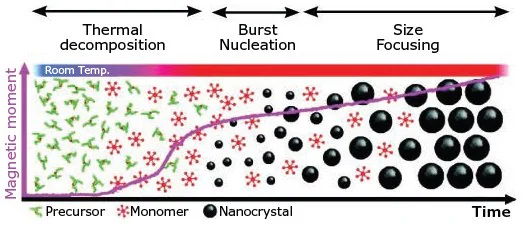
Figure 1. Schematic of the heat-up method.14 Image Credit: Merck
Typically, the precursors should exhibit no or minimal reactivity below the intended temperature to permit the predominance of growth in the target nanocrystals (highlighted in Figure 1).13
For instance, cadmium myristate, which decomposes at approximately 226 °C, and selenium powder (Prod. No. 229865), which decomposes at around 221 °C, can be used as effective precursors to generate monodisperse CdSe QD nanocrystals in octadecene (at a growth temperature of 240 °C).
Selenium powder is only soluble in octadecene at temperatures above 190 °C. Thus, research has found that the nuclei appear once the temperature reaches approximately 210 °C. This appearance is then followed by consistent growth.
Particle concentration then increases to a maximum level before subsequently decreasing.
This method effectively synthesizes high-quality and monodisperse (<5 % standard deviation of sizes) CQDs with a PLQY between 30% and 40%). A similar method, tributylphosphine telluride and octadecylphosphonate precursors, can also synthesize CdTe nanocrystals.
The Cluster-Assisted Method
The cluster-assisted method uses extremely uniform clusters or seed particles within the reaction system which act as nuclei and initiate a controlled growth of the nanocrystals.
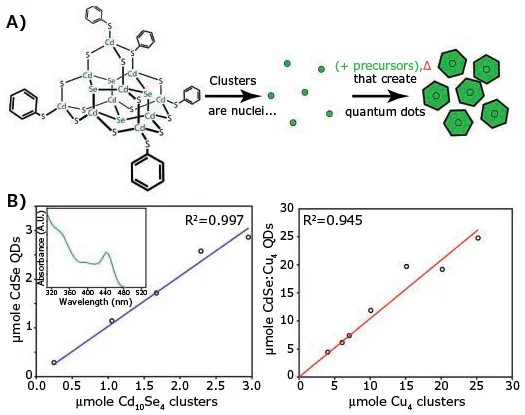
Figure 2. A) Schematic of a cluster-seed method for synthesizing quantum dots. B) Graphic of concentration of the obtained quantum dots as a function of cluster concentration.15 Image Credit: Merck
The reaction is usually instigated by combining a known concentration of a small organometallic cluster with a coordinating solvent in the presence of semiconductor precursors (as shown in Figure 2A). The temperature is then gradually increased. This reaction seeding causes the clusters to evolve into QD nucleation sites.
Snee et al. described the utilization of the cluster-seeded method to generate CdSe QDs with (NMe4)4[Cd10Se4(SPh)16] and [Na(H2O)3]2[Cu4(SPh)6] clusters.15
They utilized a standard synthesis process in which a particular quantity of the clusters was added into a mixture comprised of octadecene (7 mL) and oleylamine (1 mL) with selenium and cadmium precursors (diphenylphosphine selenide Prod. No. 802441 or tri-noctylphosphine selenide, and cadmium acetylacetonate, Prod. No. 517585).
The mixture was then stirred for 24 hours under N2 atmosphere at 50 °C. The study reported that cluster seed presence directly affected the size and concentration of the QDs. The resulting concentration of QDs was nearly identical to the quantity of cluster seeds (within 10%).
This method yielded approximately 20 times more QDs compared to a standard rapid injection procedure that utilizes an equivalent number of precursors.
Increasing the concentration of cluster seeds also decreased QD size, as the increasing number of QD nuclei competed for the limited number of available precursors. This is a flexible method of controlling QD concentration, producing a large number of QDs in a single reaction at a reduced temperature.
The Microwave-Assisted Method
The microwave-assisted method of synthesizing nanocrystals provides outstanding process control and enables higher temperatures to be reached quickly. This approach is convenient because the injection step is not necessary during the reaction and synthesis can occur under an air atmosphere.
Fluctuations in heating rate associated with conventional batch synthesis can be avoided by using a microwave.
Strouse et al. investigated the use of a microwave to synthesize nanocrystals. Microwave irradiation rapidly activated the precursors with high polarizability and could therefore be used to selectively heat the chalcogenide precursor. In doing so, Strouse and colleagues could control the growth of CdSe and CdTe nanocrystals.
Following activation, the nanocrystals undergo nucleation and development.16 In the microwave-assisted approach, the growth rate can be controlled using both microwave power and reactant concentration, while the particle size can be controlled by regulating the reaction temperature.
This method is reproducible and versatile, providing a reaction time under three minutes, a very small standard deviation (12% for CdTe and 6% for CdSe) in terms of size across ten different reactions, and emission line widths of approximately 40 nm (CdTe) and 27–28 nm (CdSe).
In a conventional microwave-assisted synthesis, cadmium stearate in an alkane solvent is mixed with the selenium powder in tri-n-octylphosphine in a microwave reaction vessel at a 1:5 molar ratio of cadmium to selenium.
Hexadecylamine (Prod. No. 445312) is introduced to the mixture and then a septum is utilized to seal the vessel. The vessel is subsequently positioned inside a microwave and heated to 240 °C with a ramping period of 30 seconds at a power of 300 W and a holding period of 30 seconds at a temperature of 240 °C.
The reaction is cooled to room temperature immediately. In a study by Kappe et al., the standard hot-injection method was compared with the microwave-assisted method. Kappe and colleagues found that QD quality was identical in both.17
The Continuous-Flow Method
The continuous-flow method provides unique benefits over batch synthesis. For instance, it offers a more feasible path to mass production.
With the continuous flow reaction approach, automating and controlling the reaction parameters is possible. This makes the optimization process more efficient, enhances the mixing of the precursors, and increases scalability.
Advancements in the reactor design employed in the continuous-flow method have provided substantial improvements regarding the automation of a large-scale chemical reaction.
Two-phase segmented-flow methods (liquid–liquid or gas–liquid) overcome the challenges of slow diffusive mixing of precursors and broad residence time distribution characteristic of the single-phase laminar flow design.
A two-phase segmented flow also improves the mixing of precursors and facilitates optimal heat and mass transfer in smaller volumes (isolated droplets) of chemical reactions. This approach has been shown to produce more monodispersed nanomaterials with higher quality.18,19
For instance, PbS CQDs can be synthesized using a dual-stage continuous-flow reactor system with a two-phase segmented-flow approach (liquid–liquid).
Growth and nucleation occur in two separate reactors when the dual-stage system is used. This enables the application of different temperatures and therefore provides more control over the nucleation process.20
Two-phase segmentation is attained by introducing an inert, immiscible fluid (Fluorinert™ FC-70, Prod. No. F9880) into every precursor under stirring conditions. Precursor A comprises lead oleate in octadecene, and precursor B comprises bis(trimethylsilyl) sulfide (Prod. No. 283134) in octadecene.
These precursors are pumped into the mixer under N2 overpressure. They subsequently advance to the nucleation reactor at the temperature of nucleation (TN), and then the growth reactor at the temperature of growth (TG). The reaction can also be controlled by adjusting the total residence time (tR) of the reaction inside each reactor.
Through the optimization of parameters (TN, TG, tR) in the dual-stage system, it is possible to synthesize high-quality PbS CQDs. The quality achieved is comparable to that of the hot-injection method but with the added advantage of greater production scalability. The standard production yield is 2.4–2.5 g/h.
Conclusions
Due to developments in synthetic methods, it is possible to synthesize monodisperse CQDs using various approaches. Many of these methods attempt to attain comparable results, in that they use similar mechanisms to yield the homogeneous nucleation and controlled growth of monodisperse CQD nanocrystals.
While the hot-injection method is the most popular approach to synthesizing monodisperse CQDs, alternative methods like cluster-assisted, heat-up, microwave-assisted, and continuous flow can provide significant benefits and versatility in terms of process efficiency.
Typically, the heat-up method enables nanocrystals to be prepared in a single pot without an injection step. The microwave-assisted method is similar but enables higher temperatures to be reached quickly, completing synthesis within minutes.
The cluster-assisted approach offers control over the concentration of QDs and allows a large amount of production. However, it requires the careful design of an appropriate seed cluster. The continuous flow-assisted method is an automated approach to synthesizing high-quality CQD nanocrystals on a production scale.
Numerous methods and synthetic tools are available for the fabrication of QDs. The method utilized depends on the materials system's temperature and chemical composition requirements, in addition to the target production scale.
References and Further Reading
- Kim JY, Voznyy O, Zhitomirsky D, Sargent EH. 2013. 25th Anniversary Article: Colloidal Quantum Dot Materials and Devices: A Quarter-Century of Advances. Adv. Mater.. 25(36):4986-5010. https://doi.org/10.1002/adma.201301947
- Talapin DV, Lee J, Kovalenko MV, Shevchenko EV. 2010. Prospects of Colloidal Nanocrystals for Electronic and Optoelectronic Applications. Chem. Rev.. 110(1):389-458. https://doi.org/10.1021/cr900137k
- Pan J, Sarmah SP, Murali B, Dursun I, Peng W, Parida MR, Liu J, Sinatra L, Alyami N, Zhao C, et al. 2015. Air-Stable Surface-Passivated Perovskite Quantum Dots for Ultra-Robust, Single- and Two-Photon-Induced Amplified Spontaneous Emission. J. Phys. Chem. Lett.. 6(24):5027-5033. https://doi.org/10.1021/acs.jpclett.5b02460
- Carey GH, Abdelhady AL, Ning Z, Thon SM, Bakr OM, Sargent EH. 2015. Colloidal Quantum Dot Solar Cells. Chem. Rev.. 115(23):12732-12763. https://doi.org/10.1021/acs.chemrev.5b00063
- Ekimov A, Onushchenko A. 1981. Quantum size effect in three-dimensional microscopic semiconductor crystals. Jetp Lett. 34(6)345-349.
- Bodnarchuk MI, Kovalenko MV. Engineering colloidal quantum dots.1-29. https://doi.org/10.1017/cbo9781139022750.002
- Kagan CR, Lifshitz E, Sargent EH, Talapin DV. 2016. Building devices from colloidal quantum dots. Science. 353(6302):aac5523-aac5523. https://doi.org/10.1126/science.aac5523
- LaMer VK, Dinegar RH. 1950. Theory, Production and Mechanism of Formation of Monodispersed Hydrosols. J. Am. Chem. Soc.. 72(11):4847-4854. https://doi.org/10.1021/ja01167a001
- Murray CB, Kagan CR, Bawendi MG. 2000. Synthesis and Characterization of Monodisperse Nanocrystals and Close-Packed Nanocrystal Assemblies. Annu. Rev. Mater. Sci.. 30(1):545-610. https://doi.org/10.1146/annurev.matsci.30.1.545
- Peng X, Wickham J, Alivisatos AP. 1998. Kinetics of II-VI and III-V Colloidal Semiconductor Nanocrystal Growth: ?Focusing? of Size Distributions. J. Am. Chem. Soc.. 120(21):5343-5344. https://doi.org/10.1021/ja9805425
- Murray CB, Norris DJ, Bawendi MG. 1993. Synthesis and characterization of nearly monodisperse CdE (E = sulfur, selenium, tellurium) semiconductor nanocrystallites. J. Am. Chem. Soc.. 115(19):8706-8715. https://doi.org/10.1021/ja00072a025
- Protesescu L, Yakunin S, Bodnarchuk MI, Krieg F, Caputo R, Hendon CH, Yang RX, Walsh A, Kovalenko MV. 2015. Nanocrystals of Cesium Lead Halide Perovskites (CsPbX3, X = Cl, Br, and I): Novel Optoelectronic Materials Showing Bright Emission with Wide Color Gamut. Nano Lett.. 15(6):3692-3696. https://doi.org/10.1021/nl5048779
- Yang YA, Wu H, Williams KR, Cao YC. 2005. Synthesis of CdSe and CdTe Nanocrystals without Precursor Injection. Angew. Chem. Int. Ed.. 44(41):6712-6715. https://doi.org/10.1002/anie.200502279
- Kwon SG, Piao Y, Park J, Angappane S, Jo Y, Hwang N, Park J, Hyeon T. 2007. Kinetics of Monodisperse Iron Oxide Nanocrystal Formation by ?Heating-Up? Process. J. Am. Chem. Soc.. 129(41):12571-12584. https://doi.org/10.1021/ja074633q
- Jawaid AM, Chattopadhyay S, Wink DJ, Page LE, Snee PT. 2013. Cluster-Seeded Synthesis of Doped CdSe:Cu4 Quantum Dots. ACS Nano. 7(4):3190-3197. https://doi.org/10.1021/nn305697q
- Washington II AL, Strouse GF. 2008. Microwave Synthesis of CdSe and CdTe Nanocrystals in Nonabsorbing Alkanes. J. Am. Chem. Soc.. 130(28):8916-8922. https://doi.org/10.1021/ja711115r
- Moghaddam MM, Baghbanzadeh M, Keilbach A, Kappe CO. 2012. Microwave-assisted synthesis of CdSe quantum dots: can the electromagnetic field influence the formation and quality of the resulting nanocrystals?. Nanoscale. 4(23):7435. https://doi.org/10.1039/c2nr32441e
- Yen BKH, Günther A, Schmidt MA, Jensen KF, Bawendi MG. 2005. A Microfabricated Gas-Liquid Segmented Flow Reactor for High-Temperature Synthesis: The Case of CdSe Quantum Dots. Angew. Chem.. 117(34):5583-5587. https://doi.org/10.1002/ange.200500792
- Mehenni H, Sinatra L, Mahfouz R, Katsiev K, Bakr OM. 2013. Rapid continuous flow synthesis of high-quality silver nanocubes and nanospheres. RSC Adv.. 3(44):22397. https://doi.org/10.1039/c3ra43295e
- Pan J, El-Ballouli AO, Rollny L, Voznyy O, Burlakov VM, Goriely A, Sargent EH, Bakr OM. 2013. Automated Synthesis of Photovoltaic-Quality Colloidal Quantum Dots Using Separate Nucleation and Growth Stages. ACS Nano. 7(11):10158-10166. https://doi.org/10.1021/nn404397d
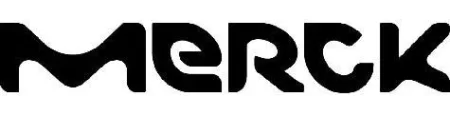
This information has been sourced, reviewed and adapted from materials provided by Merck.
For more information on this source, please visit Merck.