A group of researchers recently published a paper in the journal Science Advances that demonstrated the effectiveness of supramolecular systems developed from oligoamide sequences (OSs) in reversibly switching the plasmonic nanogaps between the quantum and classical tunneling regime.
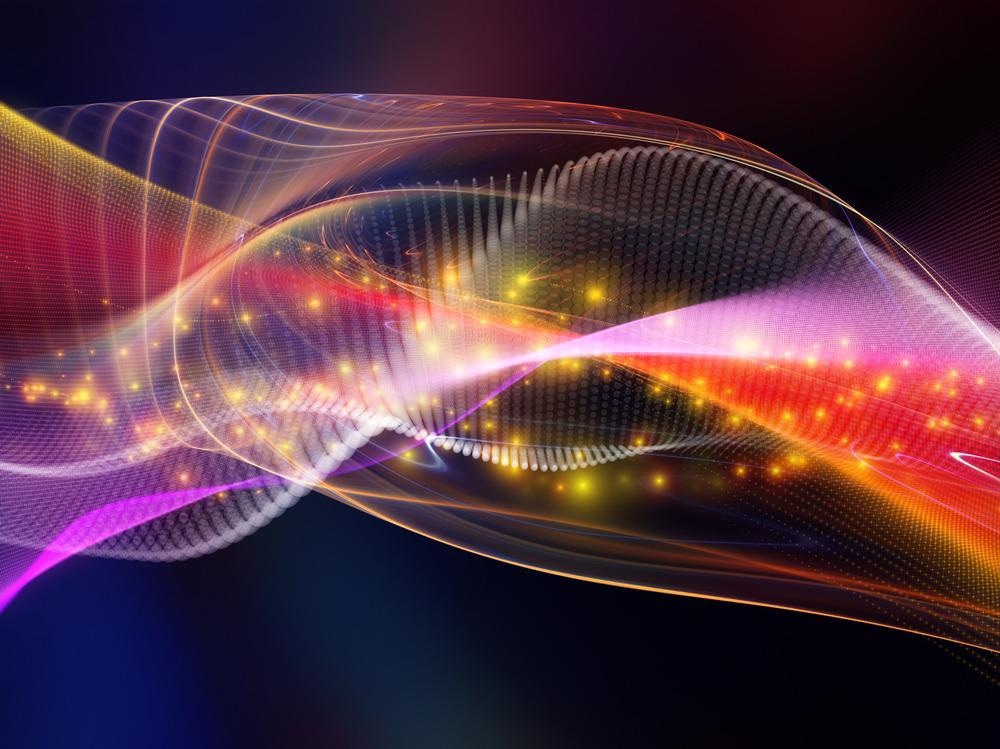
Study: Switching plasmonic nanogaps between classical and quantum regimes with supramolecular interactions. Image Credit: agsandrew/Shutterstock.com
Background
Plasmonics has quickly flourished into an interdisciplinary and vibrant field owing to its extensive range of applications such as energy harvesting and nano-optics.
In nanoplasmonics, engineering the plasmonic nanogaps/hotspots is one of the most challenging aspects as several crucial plasmonic properties are associated with their small mode volume and large electric field confinement.
Plasmonic nanogaps are extremely sensitive to gap distance that can extend to the sub picometer range. As the gap distance enters the subnanometer scale, the nonlocal screening and the quantum nature of the electrons often change the plasmonic response.
However, the lack of reversible switching of nanogaps across the quantum tunneling limit at 0.5 nm, where both quantum and classical mechanical models apply, is a significant challenge. The absence of a reversible switching system has hindered the use of quantum plasmonic devices in several applications.
Among the fabrication methods devised for reducing the sizes of nanogaps below the quantum tunneling limit, supramolecular systems with sizes at the scale of subnanometer are the most suitable as they facilitate easy access to the quantum limit.
An OS, which is an artificially designed supramolecular system that can alter its size in the angstrom range, was developed for switching the gaps in the subnanometer range across the quantum tunneling limit.
OSs are mainly composed of a fluoroquinoline-based tetramer and pyridine-based trimer and can form a single helical structure and an antiparallel double helix.
In this study, researchers investigated the viability of using supramolecular systems composed of OSs to reversibly switch the plasmonic gaps between quantum and classical tunneling regimes of gold (Au) nanoparticles on the mirror through supramolecular interactions.
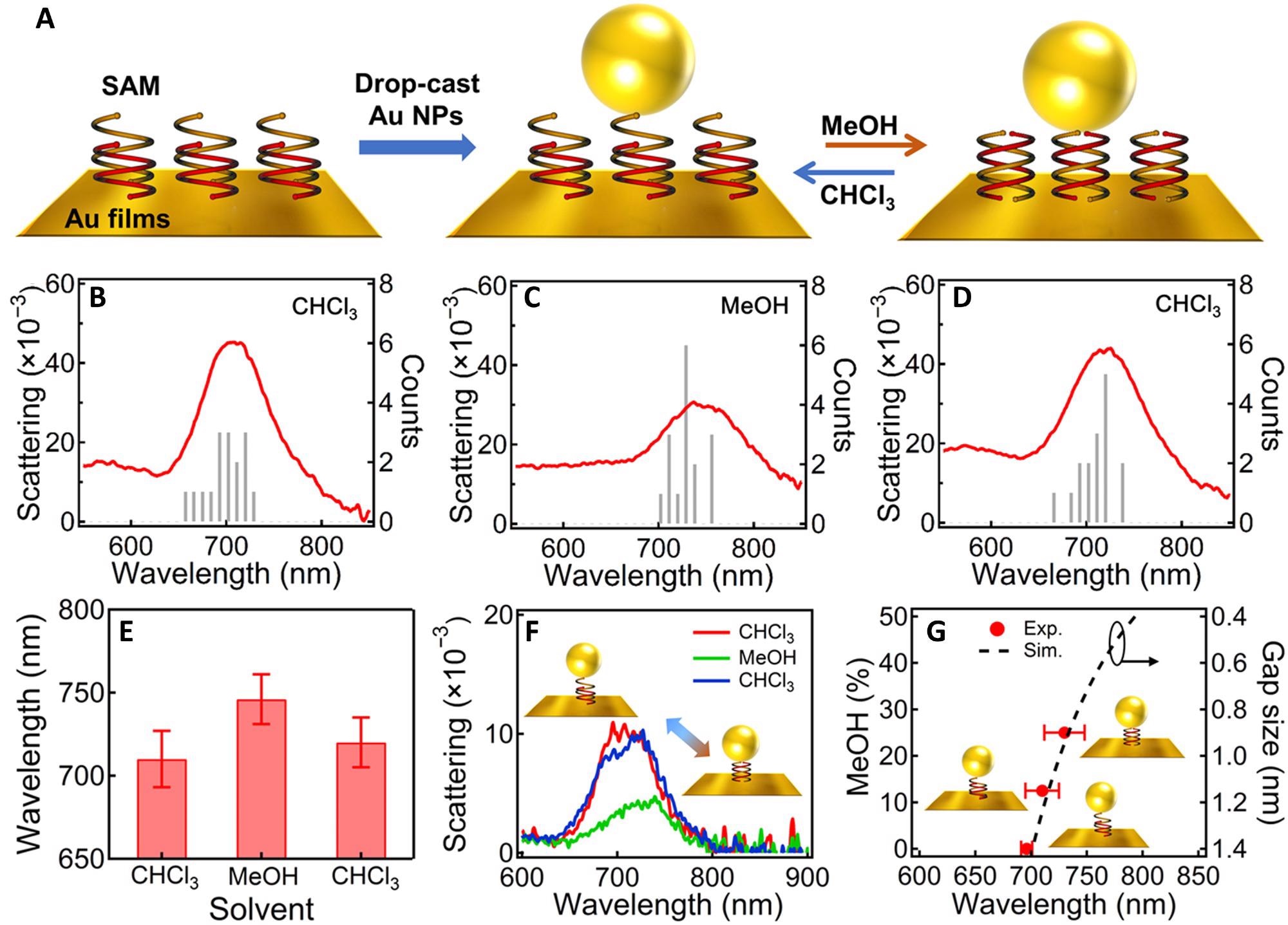
Figure 1. Solvent-induced reversible tuning of Au NPoM plasmons. (A) Scheme of the Au NPoM with OS double helices SAM in the nanogap and reversible tuning mechanism. (B to D) Statistics of the DF scattering spectra of Au NPoMs after incubating in different solvents: (B) CHCl3, (C) MeOH, and (D) CHCl3. The spectra are collected over 15 randomly selected NPs and averaged. (E) Change of the plasmon peak position after incubating in different solvents. (F) DF scattering spectra of the same particle after incubating in CHCl3, MeOH, and CHCl3 again. (G) Change of plasmon resonance with MeOH content (red dots) and simulation based on circuit model (black dashed line). © Zhang, C., Mao, L., Xu H. et al. (2022)
The Study
The compounds OS-2 and OS-1 were synthesized through acid chloride-amine condensation reactions, followed by the elimination of the amino-protecting triphenylmethyl group. Nuclear magnetic resonance spectroscopy was used to characterize the synthesized products.
A 70 nm Au film on silicon (Si) substrate was immersed overnight in 20 mM OS-2 or OS-1/chloroform solution, and then pure chloroform was used to rinse the film. Finally, the film was dried quickly. Pure chloroform, pure methanol (MeOH), and dichloromethane were mixed at the ratio of 1:8 and 1:4.
For assembly/disassembly, the sample was submerged into room temperature chloroform for 3 hours and hot chloroform at 60 oC for 30 s, respectively. All solvents were vacuum dried in order to remove the residue solvent before obtaining the dark-field (DF) scattering spectra.
A customized DF microscope with a fiber spectrometer was used to record the DF scattering spectra of every Au nanoparticle on mirror (NPoM). A 641 nm continuous wave (CW) laser was utilized to excite the Au NPoMs, and the 640 nm notch filter was employed to collect the scattering spectra at the same time.
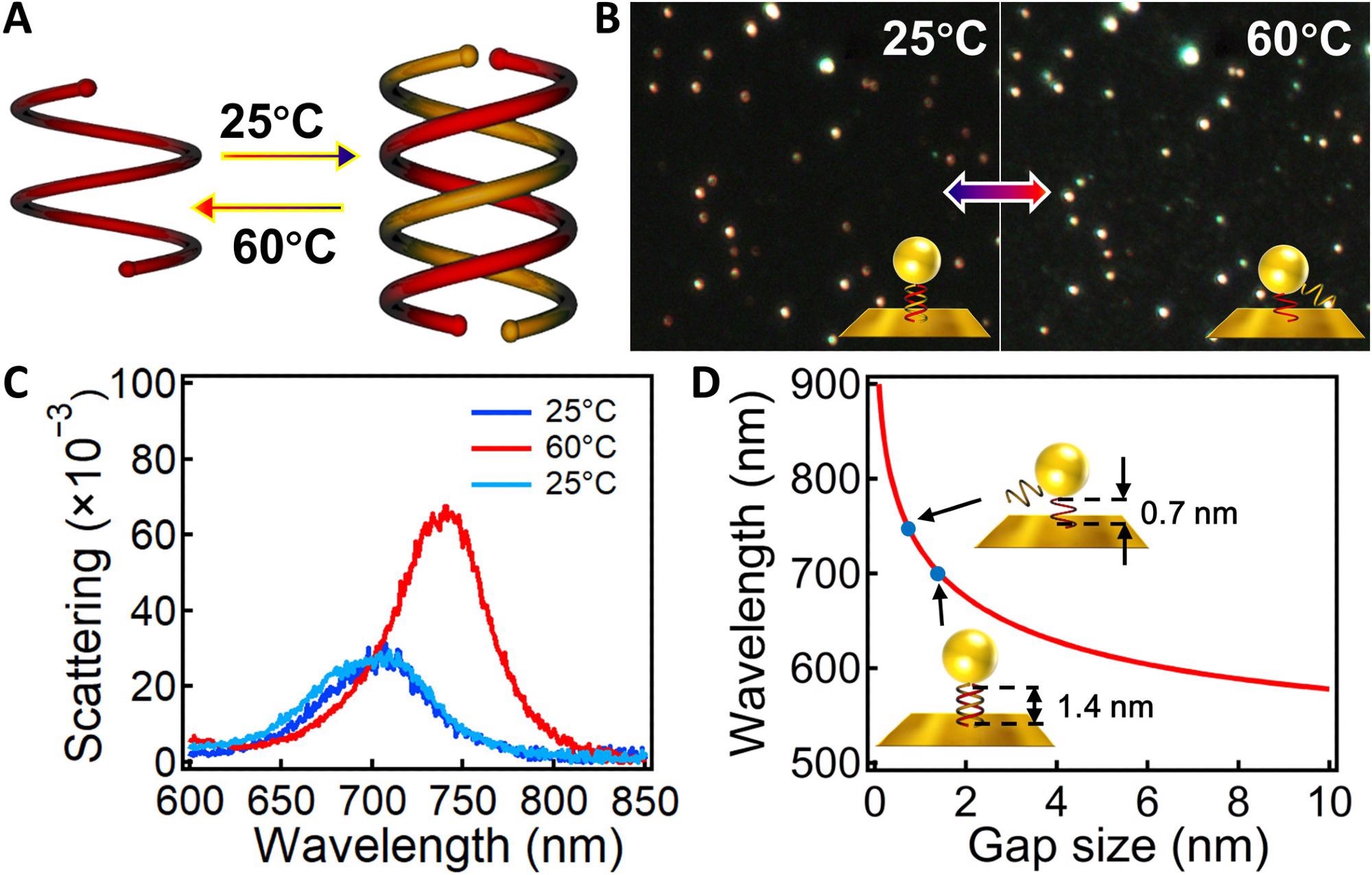
Figure 2. Temperature-induced reversible tuning of Au NPoM plasmons. (A) Scheme of the temperature-induced switching of single and double helices. (B) DF images of the Au NPoMs after incubation with CHCl3 at 25° and 60 °C for 1 hour. Insets are the schemes of the corresponding configuration of the OS assemblies in the nanogaps. (C) Scattering spectra of the same particle after incubating in chloroform for 1 hour at 25°, 60°, and then back to 25 °C. (D) Calculated change of plasmon resonance with gap size. Insets indicate the change of OS-1 in the nanogap from 1.4 to 0.7 nm after heating at 60 °C. © Zhang, C., Mao, L., Xu H. et al. (2022)
Observations
The DF scattering spectroscopy showed an average plasmon peak of 710 nm in the Au NPoMs when OS-1 compound was used, and after these NPoMs were incubated in MeOH and vacuum dried, the plasmon resonances red-shifted to 746 nm. The observations indicated that the conformational alteration of the double helix with the change of solvent was responsible for the shift.
The gap size was increased to 1.4 nm and the plasmon resonances blue-shifted to 720 nm when the samples were incubated in chloroform due to the recovery of the original state of the double helix. Similarly, scattering spectra obtained by incubating the sample in various other solvents were reversible.
A red shift of the plasmon resonances from 700 to 730 nm was observed when the gap size of the Au NPoM was tuned from 1.4 to 0.9 nm. The observation perfectly matched the predictions about plasmon resonances made by the circuit model.
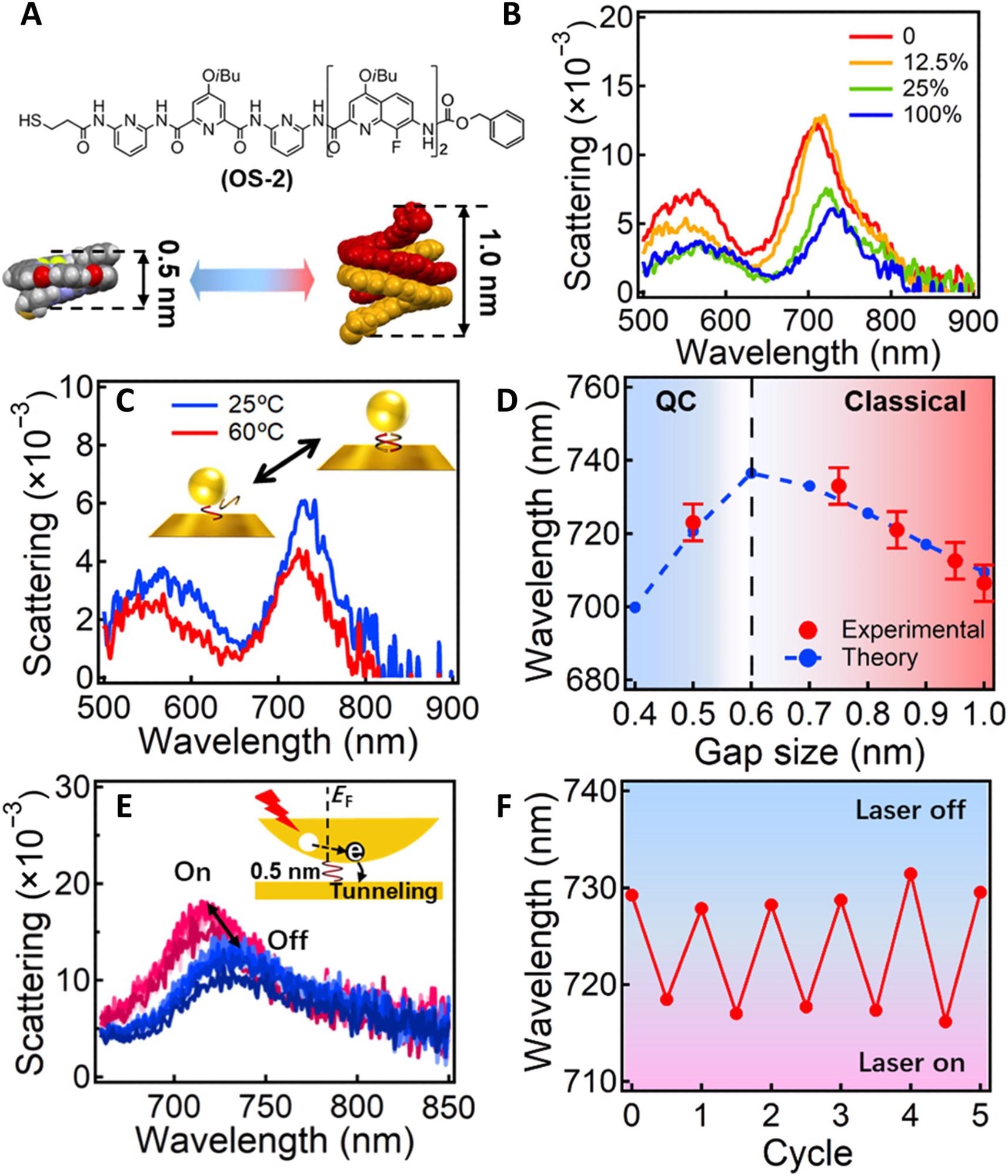
Figure 3. Tuning of the coupled plasmons of Au NPoMs across the classical and quantum regimes. (A) Formula of OS-2 and its configuration of single and double helices. (B) Scattering spectra of Au NPoM after incubating in different solvents for 1 hour. The solvents are a mixture of MeOH and CH2Cl2 with MeOH ratio increasing from 0 to 100%. (C) Scattering spectra of the same particle after incubation at 25° and 60 °C for 1 hour. (D) Change of plasmon resonance with gap size. Red dots are from the experimental, and black lines are from calculation based on quantum-corrected (QC). (E) Change of plasmon scattering peak with CW laser (641 nm, 10 μW) on and off for many cycles. Inset scheme illustrates the light-induced hot electron tunneling mechanism, which changes the conductance of the nanogap. (F) Shift of plasmon peak with 5 cycles of laser on and off. © Zhang, C., Mao, L., Xu H. et al. (2022)
The gap size was increased when double helices were disassembled into single strands due to increasing temperature and decreased with the decreasing temperature.
A reversible shift was observed in the plasmon resonance of the Au NPoM between 705 and 739 nm when temperatures switched between 25 oC and 60 oC, indicating that the complete dissociation and recombination of double helices require 30 s and 3 hours, respectively.
The plasma resonance red-shifted from 703 to 733 nm with the increasing ratio of dichloromethane and pure chloroform when OS-2 was used as the double helices were contracted due to higher π-π interactions.
However, the gap size is reduced when the double helices were fully dissociated at higher temperatures and the plasmon resonance blue-shifted to 723 nm, indicating a usual feature of quantum plasmonics. A blue shift observed in the plasmon peak after laser irradiation was reversed when the laser was switched off.
Taken together, the findings of this study demonstrated that placing the OSs in the plasmonic nanogaps can facilitate reversible switching between quantum and classical tunneling regimes and help in gaining insights on quantum plasmonic transitions.
Reference
Zhang, C., Mao, L., Xu H. et al. (2022) Switching plasmonic nanogaps between classical and quantum regimes with supramolecular interactions. Science Advances. Available at: https://www.science.org/doi/10.1126/sciadv.abj9752
Disclaimer: The views expressed here are those of the author expressed in their private capacity and do not necessarily represent the views of AZoM.com Limited T/A AZoNetwork the owner and operator of this website. This disclaimer forms part of the Terms and conditions of use of this website.