By Pritam RoyReviewed by Susha Cheriyedath, M.Sc.Nov 21 2022
In an article published in Scientific Reports, researchers investigated the slime expelled by the velvet worm (Epiperipatus biolleyi), both after and before expulsion. They also thoroughly studied the nano- and microstructures of the slime. The findings revealed abundant encapsulated carbonate salts and phosphate in addition to the previously described carbohydrates, protein nanoglobules, and lipids.
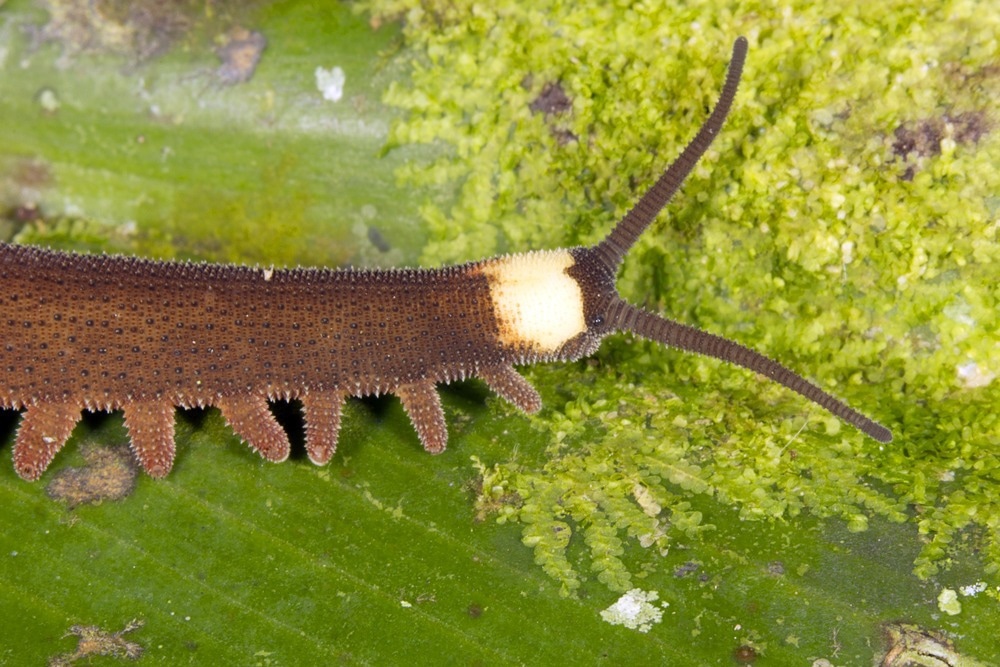
Study: Encapsulated salts in velvet worm slime drive its hardening. Image Credit: Dr Morley Read/Shutterstock.com
Additionally, CO2 bubbles were detected as the slime hardened. These results, coupled with further observation, pointed to the possibility that the encapsulated salts from the expelled slime quickly neutralized and dissolved in a manner analogous to a baking-powder reaction, which quickened the drying of the slime. Shear stress and the ions’ neutralization reaction consequently affected the proteins’ aggregation and conformation, raising the ionic strength and pH of the slime.
These findings about the drying procedure of the expelled slime of the velvet worm demonstrated how naturally formed polymerizations could unravel in seconds. The results also inspired novel polymers that were fast-drying or stimuli-responsive under suitable conditions.
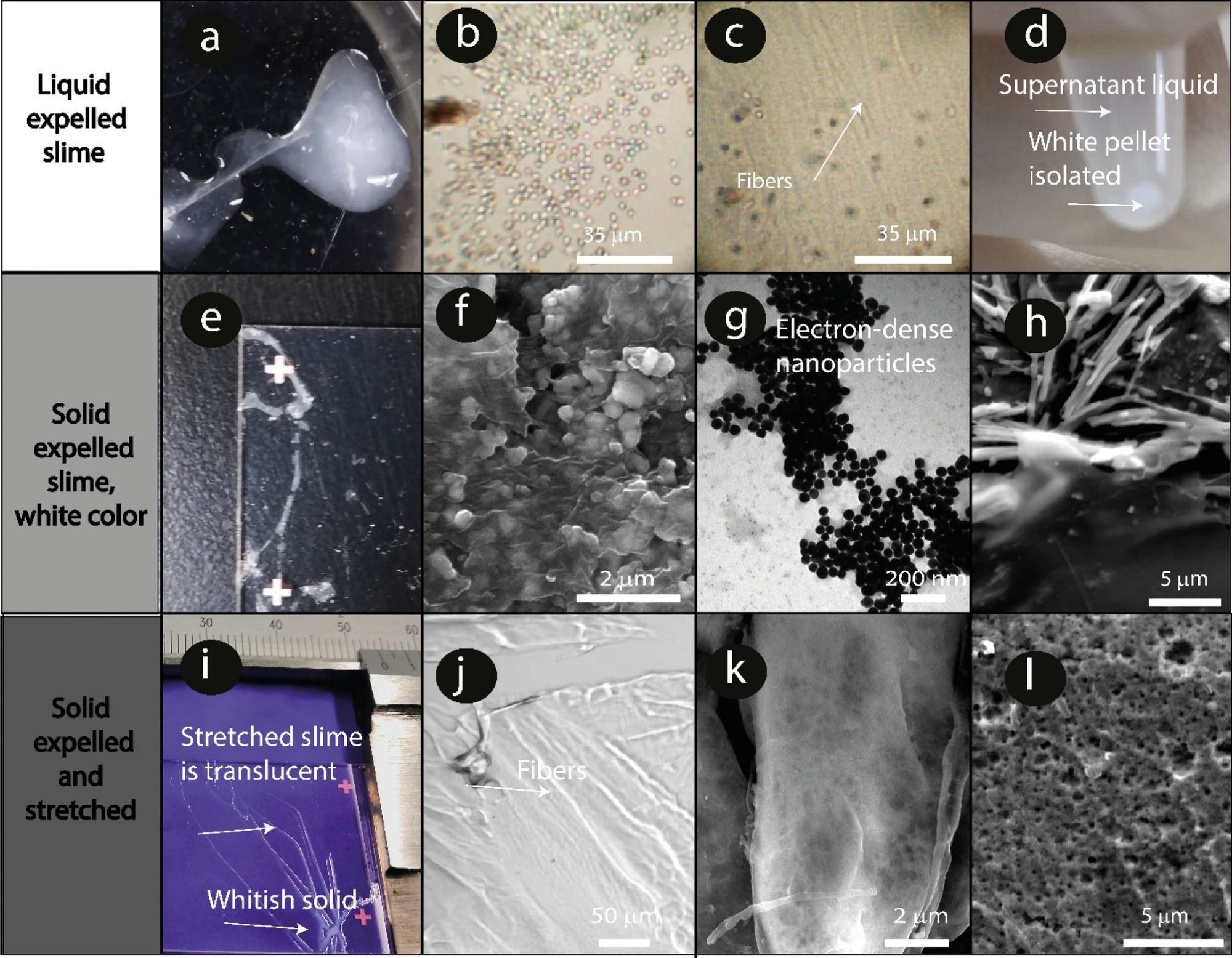
Figure 1. (a-d): Liquid expelled slime, (e–h): solid expelled slime, (i-l) solid expelled slime after a mechanical stimulus (stretching). (a) Photograph of the white expelled slime on a polystyrene Petri dish. Note that the slime remains liquid without external mechanical stimuli such as stretching. The sample was handled carefully to avoid shear stress. (b) Bright-field image of the dispersed roundish-microparticles in the liquid protein slime matrix. (c) Bright-field image of the fibers and microparticles dispersed in the liquid slime. (d) White pellet precipitated from the viscous white slime solution. Note that the liquid supernatant is translucent. (e) Photograph of the dried expelled slime that did not experience any external mechanical stimulus on a glass slide. Note that this slime remained white. (f) SEM micrograph of nano- and microparticles dispersed in the slime. (g)TEM image of electron-dense roundish nanoparticles < 500 nm size. (h) SEM image of the fibers and microparticles in the solid expelled slime. (i) Expelled slime on a glass slide. Note that the slime in the upper part of the image is translucent (it was stretched) and that the unstretched slime in the lower part remains white. (j) Fibers observed in the translucent area (k) SEM image of the slime threads formed. Note the absence of nano- and microparticles. (l) SEM cross-section image of one slime thread. A porous structure is present.
Velvet Worm Slime and Its Potential in Biomedical and Technological Breakthroughs
The requirement for considerable adherence to delicate tissues, even in damp environments, presents a difficulty to biomedical adhesives. Knowledge-based material advancement can be inspired by biological materials and processes from natural sources that permit tailoring adhesion. Thus, such tailored adhesion indicates potential biomedical and technical advancements, for example, in the applications of 3D bioprinting of biomacromolecules.
According to this perspective, the distinctive slime secretion utilized by the velvet worm (an invertebrate of the phylum Onychophora) for prey capture, defense, and parental feeding, is an excellent example. The sticky, white slime that the Central American velvet worm secretes attaches tenaciously to a wide range of materials, including metals, wood, biological tissues, and glass.
Prior investigations on the slime-ejecting process of the velvet worm used high-speed videography and anatomical images. The muscles around the slime reservoir of the worms’ syringe-hose system contract, propelling the slime through a funnel-shaped channel at a higher fluid velocity. This slime then flows through two oral papillae that oscillate at a frequency of around 30 hertz. The slime flow creates two slime jets that produce a sticky fiber mesh surrounded with droplets of liquid that are a few micrometers in size.
The physicochemical mechanism that causes the quick drying of the slime following ejection and mechanical stimulation remains unexplained, although the secreted slime has already been partially characterized. This discharged slime is a viscous liquid after ejection. Still, in response to mechanical stimuli, such as the movements of a trapped insect, it quickly changes into a highly expanding rubber-like material before becoming a hard glassy material. This liquid-to-solid transition happens as fast as 10 seconds to one minute after ejection and mechanical stimulation.
The electron-dense particles in the velvet worm slime were physicochemically characterized in the current study. Changes in the slime structure and its characteristics were also examined. The authors described the ejected slime before and after introducing an external mechanical stimulus. Also, the unexpelled slime extracted from the reservoir in situ was characterized. Finally, the nano and microparticles and the hemolymph extracted from the slime were analyzed to rule out artifacts caused by contamination.
Several methods, including cryo-transmission electron microscopy (cryo-TEM), energy-dispersive X-ray spectroscopy (EDS), and scanning transmission electron microscopy (STEM), among others, were used to examine the slime nano and microparticles. Inductively Coupled Plasma Spectroscopy (ICP) and EDS were used to compare the chemical structure of the hemolymph and slime.
Finally, atomic force microscopy (AFM) and mechanical dynamical analysis (DMTA) were used to investigate how the salts affected the slime’s Young’s modulus and the tension pull-off adhesion force among two glass blocks.
Proof-of-Concept Investigation
Epiperipatus biolleyi specimens were gathered from Las Nubes de Coronado, Costa Rica. 10 female velvet worms were dissected. The unexpelled, liquid slime was then allowed to flow out of the slime reservoir once it was removed and opened. Characterization analyses were completed right after the slime was collected to prevent bacterial development.
The fluorescence staining of nano and microstructures was conducted within the ejected slime and isolated particles. Several dyes were used to stain these nano and microstructures in the ejected slime and separated particles, including rhodamine B isothiocyanate.
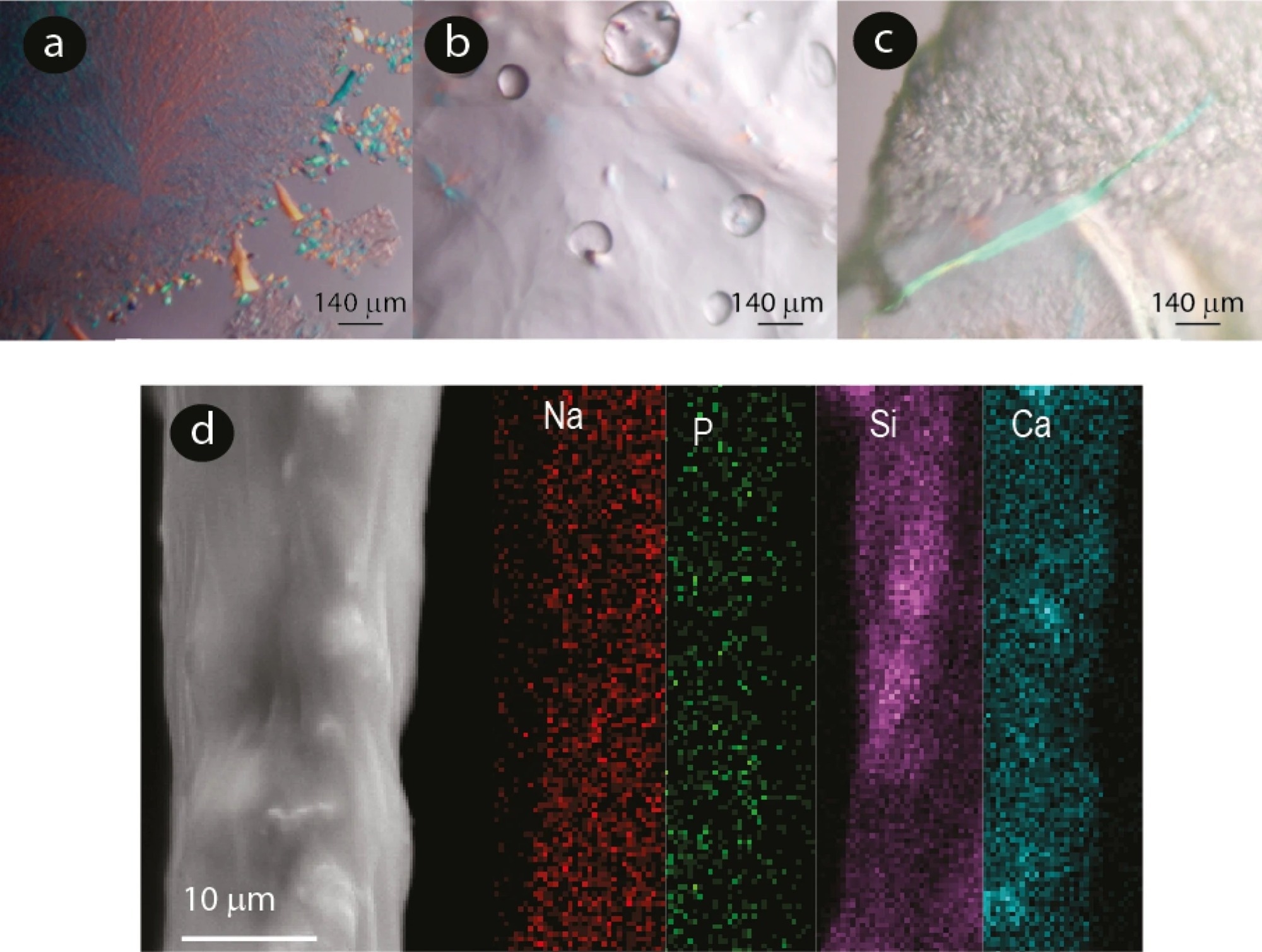
Figure 2. Microscopy images of slime. (a) Polarized microscopy images of slime expelled before experiencing an external mechanical stimulus showing crystalline structures. (b) and (c) slime expelled after an external mechanical stimulus. (d) SEM micrograph of an expelled slime fiber and elemental mapping using EDS.
Velvet worm slime was collected and characterized using high-resolution microscopy and optical analysis. The expelled liquid slime was a white liquid that had no glassy aspect. When the velvet worm slime was allowed to pass into an Eppendorf tube and subsequently centrifuged, a white pellet comprising nano and microparticles was produced.
Compared to expelled slime not subjected to an external mechanical stimulus, the slime immediately extracted from the reservoir exhibited similar characteristics, including white color, fibers, and dispersed nano and microparticles in suspension. These results indicated that the crystalline nano and microparticles were not a drying artifact, a slime expulsion byproduct, or an external contaminant.
Multiple lines of evidence indicated that the nano and microparticles in the velvet worm slime previously stabilized by a lipid or protein covering disintegrated and reacted with one another in the acidic slime matrix both after and during the expulsion process and mechanical stimuli. Natron and nahpoite’s chemical identities were accurately determined by analyzing the crystal diffraction signals.
The enhanced pH and ionic strength favored the assembly and connections of the coextensive fibrous proteins. Along with improving the glassy look and gel-like behavior of the velvet worm slime, the low molecular weight compounds and the distributed salts also improved its adhesion force and Young’s modulus.
Significance of the Study
The results generated by a time-dependent and high-resolution physicochemical characterization showed that mechanical stimuli influenced the chemical and structural changes in the velvet worm slime. A microstructural transformation that started with globules and aggregates comprising particles high in carbon (C), oxygen (O), phosphorous (P), sodium (Na), calcium (Ca), and chlorine (Cl) before the expulsion correlated with changes in pH value and the visual features (turbid to transparent).
In general, the findings suggested a multi-stage process for the hardening and gelation of the velvet worm slime. The reservoir contained the slime in a liquid-crystalline condition with an acidic pH. During ejection, the phosphate and inorganic carbonate particles dispersed, indicating that they reacted with one another and the liquid slime. Thus, it elevated the pH level of the ejected slime, generated CO2, and enhanced the ionic strength.
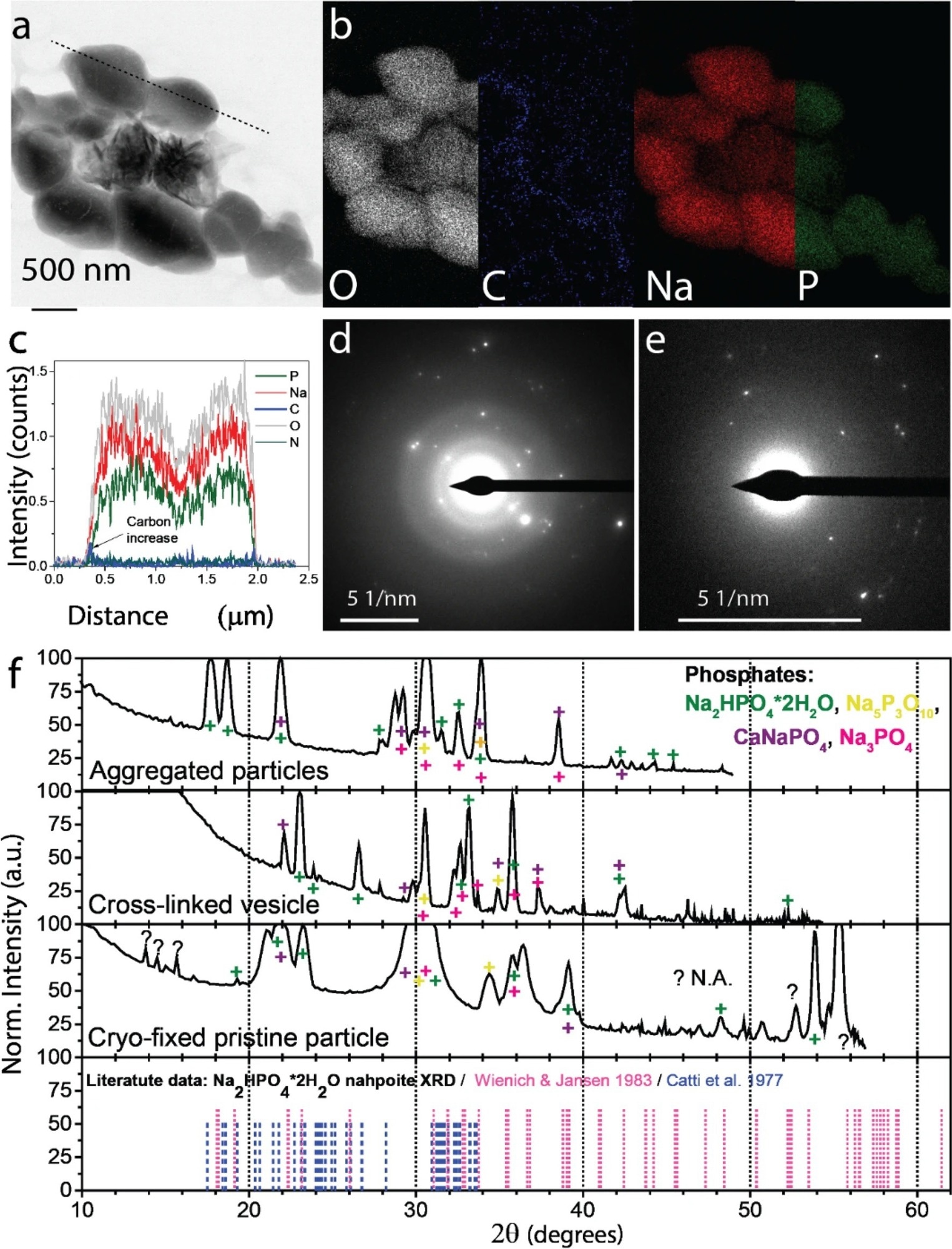
Figure 3. Morphology and chemical composition of phosphate particles in the expelled slime before experiencing an external mechanical stimulus. (a) STEM , BF micrograph of isolated polyphosphate globule containing angular phosphate particles. (b) Elemental mapping of particles shown a (STEM-EDSX). Elemental mappings of less abundant elements are presented in Supplementary Fig. 18. (c) Elemental profile of the dotted line in panel (a). Cryo-TEM-selected area electron diffraction (SAED) analysis of (d) phosphate particle precipitated from the expelled slime before experiencing an external mechanical stimulus, (e) aldehyde-fixed phosphate particles aggregate. (f) Crystalline phase analysis based on chemical composition and d-spacings. The color symbols show peaks corresponding to different phosphate species.
Following these variations in the slime, the protein aggregation, shear stress, and phase separation led to the hardening and drying of the slime, resulting in the development of a robust composite biopolymer. The quick polymer curing via lipid-protein-stabilized phosphate and carbonate microparticles proposed in this paper might be utilized to design novel industrial polymerization procedures where aqueous formulation of polymers served as biocompatible adhesives or employed in intravital bioprinting.
Reference
Corrales-Ureña, Y. R et al. (2022). Encapsulated salts in velvet worm slime drive its hardening. Scientific Reports. https://www.nature.com/articles/s41598-022-23523-z
Disclaimer: The views expressed here are those of the author expressed in their private capacity and do not necessarily represent the views of AZoM.com Limited T/A AZoNetwork the owner and operator of this website. This disclaimer forms part of the Terms and conditions of use of this website.