Generating electricity from natural water flow through engineered nanochannels is a viable way to address the fast-growing renewable power needs. Unfortunately, nanochannels incorporation and the multi-variable coupling's restraint on electricity production remain significant hurdles for large-scale industrial applications.
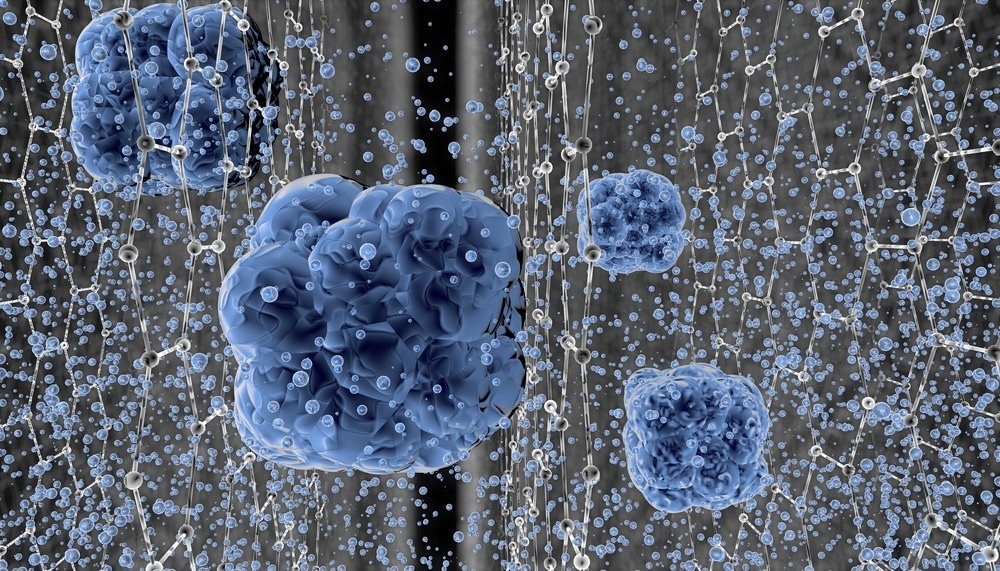
Study: Transfer learning enhanced water-enabled electricity generation in highly oriented graphene oxide nanochannels. Image Credit: Angel Soler Gollonet/Shutterstock.com
A recent study published in the journal Nature Communications addresses this problem by constructing highly aligned graphene oxide nanochannels for sustainable energy production using a freeze-casting process. This novel design encourages spontaneous absorption and directed transfer of water within the nanochannels to generate clean electricity.

Figure 1. a A 2D-WEG based on the GO assembled framework with massive integrated 2D channels can generate considerable electric energy by the spontaneous internal water flow. b To solve the multi-parameters coupling optimization problem of the developed 2D-WEG, the TL framework is implemented to achieve accurate prediction and optimization of generation performance using limited experimental data and abundant streaming potential data with similar backgrounds. c Ultimately, the TL-optimized 2D-WEGs with mechanical flexibility and enhanced power generation performance is produced to power a variety of commercial appliances.
Water Flow-Enabled Electricity Generation (WEG): Why is it Important?
Generating renewable energy from natural processes, including moisture dispersion, heat exchange, water flow, and evaporation, has received a lot of attention in recent years. The clean energy produced by these techniques can provide long-term electricity to the world's rapidly expanding human civilization.
Some compounds, such as molybdenum disulfide and metal-organic frameworks with artificial nanochannels, have lately exhibited promising electricity production capabilities when water runs through them because of their biocompatible and specific ion transport characteristics.
These water flow-enabled electric generators (WEGs) use a double layer of electricity at the solid-liquid interface and interfacial charge isolation caused by water flow. Moreover, the capacity to convert energy may also be increased by adjusting inherent or structural properties such as surface charge density and the size of nanochannels.
Challenges Associated with Water-Enabled Electricity Generation
Numerous significant obstacles remain in developing high-efficiency water flow-enabled electric generators (WEGs), restricting their large-scale applicability.
Even though microscopic WEGs have a high energy density, the capacity to generate energy after incorporating nanochannels into larger assemblies is problematic due to chaotic topologies and loss of coordination between isolated nanochannels.
In combination with the large-scale and homogeneous incorporation of nanochannels, the effectiveness of WEG is heavily reliant on the management of nanochannel charge density, relationships between water and substances, and intrinsic water movement. Unfortunately, the many components are tightly coupled, and any modification in one of them significantly impacts the matched configuration and total power-generating capability.

Figure 2. a Schematic illustration of the TL framework. A source model with an encoder-decoder architecture was first trained using streaming potential data. Then, the Encoder is transferred to the opt-model to initialize the parameters, and the experimental data are used to train decoding predictions from the latent space to 2D-WEGs performance. b Schematic illustration of the iterative optimization process. Initially, the generation performance data of 2D-WEGs with different structural parameters are collected experimentally. The newly collected data and the previous data are then used to refine the opt-models. Subsequently, a differential evolution algorithm is used to obtain several new combinations of structural parameters based on the predictions of opt-models, and reconstruct 2D-WEGs, and collect experimental data accordingly.
The interaction of huge nanochannels causes multi-objective trade-offs, multiparameter couplings, and extensive variable exploration, making investigations hard, costly, and time-consuming.
A substantial water pressure differential is needed on the two opposing sides of the nanochannels to enhance water flow through the nanochannels of WEGs, making the power conversion system complicated and distant from the predicted clean energy transfer mechanism.
Graphene Oxide Nanochannels for WEG Applications
Layer-by-layer assembly of 2D graphene oxide (GO) layers rich in oxygenated functional groups results in desired and directed structures. Meanwhile, when interacting with water, the variable alteration of functional groups on GO sheets may influence the surface charge density.
As a consequence, numerous and designable 2D nanochannels between graphene oxide layers may be consistently incorporated into macroscopic structures, making them suitable platforms for the creation of high-performance WEGs.
In this study, the researchers created a water flow-enabled electricity generator with 2D incorporated graphene oxide nanochannels (2D-WEG) using a rotating freeze-casting process. This 2D-WEG with customizable internal chemical and structural properties can capture water naturally and stimulate water flow within nanochannels to create considerable electrical power.
In addition, given little experimental data, the researchers used a transfer learning (TL) technique to handle the challenging multiparameter coupling adjustment for the 2D-WEG.

Figure 3. a Schematic of a waterscape screen generating electricity by spontaneous water transport. b Optical photograph of nineteen LEDs powered by a waterscape screen with 10 integrated 2D-WEGs and the inset b shows 19 LEDs being lit up. c The Voc and Isc are generated by the waterscape screen with 10 integrated 2D-WEGs when the bottom is in contact with water. d Schematic illustration of electrical output produced by watering a small architectural landscape designed by a 2D-WEG. e A commercial calculator is driven by a small architectural landscape made from a single 2D-WEG. f The Voc and Isc generated by watering the architectural landscape. g Voltage–time curves of commercial capacitors with varying capacitance charged by waving a fan made up of ten 2D-WEGs. Inset g is an optical photograph of the mechanical device that automatically waves the fan with integrated 2D-WEGs to collect electrical energy. h The electric energy stored in the capacitor power the electronic ink screen to play several animations. Source data are provided as a Source Data file.
Important Findings of the Research
In contrast to previously published single-parameter studies, the TL technique utilized in this work can give homogeneous multiparameter simulation and extremely accurate efficiency prediction even with a limited experimental dataset.
Consequently, the as-prepared 2D-WEG has remarkable mechanical adaptability and can create a high voltage of 2.9 V or a current of 16.8 A, as well as controllably change the output efficiency to meet practical needs. A simple parallel or series connection of 2D-WEGs may boost power production to 11.9 V and 82.7 A, respectively.
Additionally, the researchers created a variety of water-enabled electricity-generating devices, such as the waterscape display, the architectural panorama, and the 2D-WEG fan. These WEG systems can power scientific calculators, LED panels, and computerized ink displays, highlighting the material's potential for future clean energy development.
Based on these results, it is reasonable to conclude that the as-fabricated 2D-WEG and TL optimization techniques have considerable promise for constructing a viable water-enabled energy system.
Reference
Yang, C. et al. (2022). Transfer learning enhanced water-enabled electricity generation in highly oriented graphene oxide nanochannels. Nature Communications. Available at: https://doi.org/10.1038/s41467-022-34496-y
Disclaimer: The views expressed here are those of the author expressed in their private capacity and do not necessarily represent the views of AZoM.com Limited T/A AZoNetwork the owner and operator of this website. This disclaimer forms part of the Terms and conditions of use of this website.