The crops we eat, process, or feed to livestock are made up of micronutrients contained within soil. These micronutrients deliver the core for suitable growth and development of plants, which are consumed by them and then passed up the food chain to finally reach humans.
The balance of the soil micronutrients is of importance to all producers, growers, and consumers in the world. Soil is varied in its elemental makeup depending on the local geology and whether the soil has been altered with fertilizers or other additions throughout its use.
There is no way to completely understand the condition of the soil, make adjustments to the soil, or efficiently choose the crop to be grown in the soil, without carrying out direct elemental analysis.
The soil analysis for elemental contents is challenging during the sample preparation step. A common method for making a soil sample for inorganic elemental analysis involves digesting the soil sample in an acid that is heated to near-boiling temperature for extracting the elements for analysis.
It takes about four hours or more for this extraction method to be completed when open vessels are used in heating blocks. Then, the sample must be centrifuged or filtered for removal of solid particles before analysis. The acid digestion can be completed in less than 50 minutes using a microwave digestion system.
In addition, whenever desired, the microwave digestion system delivers complete sample digestion with the use of appropriate acids, providing a total elemental content analysis rather than only the extractable concentrations.
Inductively coupled plasma optical emission spectroscopy (ICP-OES) is generally favoured in a multi-element analytical environment with detection capabilities appropriate for the quantification of micronutrients as shown in this application. A suitable alternative is the Flame atomic absorption (AA) system, which saves costs, and provides simplicity and single-element analytical speed1.
However, measuring multiple elements with the Flame AA system requires individual analysis of each sample for each element, which can nullify the speed advantage of the Flame AA technique for multi-element analysis.
This article focuses on the analysis of micronutrients in a selection of soil samples using a PerkinElmer Avio™ 200 ICP Optical Emission Spectrometer.
Experimental
Samples and Sample Preparation
Soils can vary considerably across regions, and can differ even within a single large plot of land. To account for this variance, soil samples were collected from residential backyards and garden plots and also from pastures and commercial farms.
The garden plot samples represented highly amended and well-tended soils with a high ratio of industrially composted “bagged” soil, as generally available at garden and home stores. All soil samples symbolized healthy areas in current production and did not show any sign of depletion or disease before collection.
Two certified reference materials were used to check the accuracy of the methodology - Soil Solution A and Soil Solution B (High Purity Standards™, Charleston, South Carolina, USA). These two samples were directly analyzed, without dilution.
A micronutrient extraction method was chosen for sample preparation as this denotes the usual method for soil sample analysis. A mixture of hydrochloric acid (HCl) and nitric acid (HNO3) was used for the extraction and, predictably, residue remained for each sample following digestion.
Soil samples were then prepared for analysis by closed-vessel microwave-assisted digestion using a PerkinElmer Titan MPS microwave digestion system. Tables 1 and 2 list the sample parameters, digestion method, and the reagents used. Samples were weighed and placed into Titan MPS 75 mL Standard Pressure Digestion Vessels, and subsequently, the reagents and sample spikes, if any, were added to the samples.
Then, the samples and reagents were kept open in the vessels for 10 minutes, which allows any early reactions to take place in a safe manner. The vessels were then closed and kept in the Titan MPS system for heating and digestion.
Once the digestion was completed, the samples were shifted out of the digestion vessels by triple-rinsing with deionized (DI) water into sample vials and then diluted to the final volume. Post-dilution, the samples were left undisturbed to allow for the suspended particles to settle down. This can also be achieved quickly by centrifuging the samples for about 10 minutes.
Table 1. Titan MPS Digestion Method.
Step |
Temp (°C) |
Pressure Limit (bar) |
Ramp Time (min) |
Hold Time (min) |
Power Limit (%) |
1 |
150 |
35 |
5 |
5 |
80 |
2 |
195 |
35 |
2 |
20 |
100 |
3 |
50 |
35 |
1 |
15 |
0 |
Table 2. Digestion Information.
Parameter |
Volume |
Reagents Used |
6 mL of HCl (37%) and
3 mL of HNO3 (70%) |
Initial Sample Weight |
1 g |
Final Solution Weight (after dilution) |
50 g |
Instrumental Conditions
The analyses were performed on an Avio 200 ICP Optical Emission Spectrometer fitted with a PerkinElmer S10 Autosampler. Tables 3 and 4 outline the elements of interest and instrument conditions for the analysis of the soil samples.
A Meinhard® glass nebulizer was used with the standard baffled cyclonic spray chamber and a reduced sample uptake rate of 0.80 mL/min. The total liquid flow rate for the nebulizer, when combined with the online flow of the internal standard, was 1 mL/minute of solution.
External calibration standards were created from stock standards and were diluted with trace-metal-grade HCl and HNO3 and DI water to the final elemental concentrations provided in Table 5. The final acid concentration of the standards was about 10% of the acid blend to match the somewhat high concentration of acid in the digested and diluted samples.
Table 3. Avio 200 ICP-OES Instrumental Parameters.
Parameter |
Value |
Nebulizer |
Meinhard® Glass Type K1 (Part No. N0777707) |
Spray Chamber |
Glass Cyclonic Baffled (Part No. N0791352) |
Sample Uptake Rate (mL/min) |
0.80 |
RF Power (Watts) |
1500 |
Nebulizer Gas (L/min) |
0.70 |
Auxiliary Gas (L/min) |
0.2 |
Plasma Gas (L/min) |
8 |
Table 4. Method Parameters.
Element |
Wavelength (nm) |
Plasma View |
Integration Range (sec) |
Al |
308.215 |
Radial |
0.1-2 |
Ba |
233.527 |
Axial |
0.1-5 |
Ca |
317.993 |
Radial |
0.1-2 |
Co |
228.616 |
Axial |
0.1-5 |
Cu |
327.393 |
Axial |
0.1-5 |
Fe |
238.204 |
Radial |
0.1-2 |
K |
766.490 |
Radial |
0.1-2 |
Mg |
285.213 |
Radial |
0.1-2 |
Mn |
257.610 |
Radial |
0.1-2 |
Na |
589.592 |
Radial |
0.1-2 |
Ni |
231.604 |
Axial |
0.1-5 |
P |
178.221 |
Axial |
0.1-5 |
S |
181.975 |
Axial |
0.1-5 |
V |
292.464 |
Axial |
0.1-5 |
Zn |
206.200 |
Axial |
0.1-5 |
Y (int std) |
371.029 |
Radial |
0.1-5 |
Y (int std) |
371.029 |
Axial |
0.1-5 |
Table 5. Calibration Standards.
Element |
Std 1 (mg/L) |
Std 2 (mg/L) |
Std 3 (mg/L) |
Std 4 (mg/L) |
Std 5 (mg/L) |
Al |
|
|
25 |
100 |
500 |
Ba |
1 |
10 |
25 |
|
|
Ca |
|
|
25 |
100 |
500 |
Co |
1 |
10 |
25 |
|
|
Cu |
1 |
10 |
25 |
|
|
Fe |
|
|
25 |
100 |
500 |
K |
|
|
25 |
100 |
500 |
Mg |
|
|
25 |
100 |
500 |
Mn |
1 |
10 |
25 |
|
|
Na |
|
10 |
25 |
100 |
|
Ni |
1 |
10 |
25 |
|
|
P |
|
10 |
25 |
100 |
|
S |
|
10 |
25 |
100 |
|
|
V |
1 |
10 |
25 |
|
|
Zn |
1 |
10 |
25 |
|
|
Analysis was performed using standard 2-point background correction without using other spectral correction formulas. Yttrium was included as an internal standard for all elements analyzed using a radial or axial plasma where appropriate.
The Avio 200 spectrometer provides a number of benefits from its unique capabilities by leveraging PerkinElmer’s proven track record of ICP performance. The patented Flat Plate™ plasma technology provides a robust plasma with zero maintenance and does not require cooling while using almost half the argon plasma gas of helical load-coil systems.
The complete sample introduction system and torch assembly are combined in a single cassette for simplicity of use and maintenance. The Avio’s Dual View capability allows automated radial or axial viewing, and with its outstanding optical resolution, provides a large linear dynamic range and better detection limits and stability. All instrument control, data processing, and sample analysis were done through PerkinElmer’s Syngistix™ software.
Results and Discussion
Table 6 shows the calibration results. The excellent correlation for the setting standards shows the precision and accuracy of the Avio 200 ICP-OES. The independent calibration verification (ICV) recoveries confirm the validity of the calibration and the accuracy of the creation of the standards.
Table 6. Calibration Results.
Element |
Correlation Coefficient |
ICV (% Recovery) |
Al |
0.99999 |
97 |
Ba |
0.99999 |
98 |
Ca |
0.99998 |
101 |
Co |
0.99993 |
96 |
Cu |
0.99988 |
96 |
Fe |
0.99999 |
100 |
K |
0.99992 |
97 |
Mg |
0.99991 |
108 |
Mn |
0.99999 |
102 |
Na |
0.99985 |
96 |
Ni |
0.99998 |
97 |
P |
0.99986 |
98 |
S |
0.99985 |
98 |
V |
0.99995 |
99 |
Zn |
0.99990 |
98 |
To verify the accuracy of the methodology, two certified reference material soil solutions were measured; their results are shown in Table 7. All concentrations are within 15% of the certified values for the two samples, confirming the accuracy of the method.
The total sample-to-sample analysis time is less than 5 minutes for the measurement of 15 elements and internal standards. By comparison, a typical Flame AA instrument would take more than 30 minutes to sequentially analyze the same list of elements, not including the required extra calibration time.
Table 7. Analysis of Soil Reference Solutions.
Element |
Soil Solution A |
Soil Solution B |
Certified (mg/L) |
Experimental (mg/L) |
% Recovery |
Certified (mg/L) |
Experimental (mg/L) |
% Recovery |
Al |
500 |
459 |
92 |
700 |
662 |
95 |
Ba |
5 |
4.75 |
95 |
7.00 |
6.94 |
99 |
Ca |
350 |
343 |
98 |
125 |
126 |
101 |
Co |
--- |
0.027 |
--- |
0.100 |
0.087 |
87 |
Cu |
0.300 |
0.289 |
96 |
3.00 |
3.01 |
100 |
Fe |
200 |
201 |
101 |
350 |
356 |
102 |
K |
200 |
196 |
98 |
210 |
210 |
100 |
Mg |
70 |
73 |
104 |
80.0 |
82.6 |
103 |
Mn |
0.100 |
0.110 |
110 |
100 |
95.2 |
95 |
Na |
70.0 |
63.8 |
91 |
100 |
92.5 |
92 |
Ni |
0.300 |
0.287 |
96 |
0.20 |
0.20 |
100 |
P |
--- |
6.72 |
--- |
--- |
6.76 |
--- |
S |
--- |
1.86 |
--- |
--- |
2.03 |
--- |
V |
0.100 |
0.096 |
96 |
0.800 |
0.772 |
97 |
Zn |
1.00 |
1.02 |
102 |
70.0 |
68.9 |
98 |
After the analytical methodology is validated, the collected soil samples were prepared and analyzed. Using the Titan MPS digestion system, the samples were easily and quickly prepared for analysis, saving time significantly over the typical process of open-vessel digestion.
Figure 1 shows the results from the analysis of the soil samples and demonstrates the value of quantitative analysis in the characterization of samples. Some soils varied by as much as a factor of 10x in the concentration of certain elements, but otherwise exhibited unexpected consistency. This is entirely expected since all the samples were collected from a relatively small geographic area.
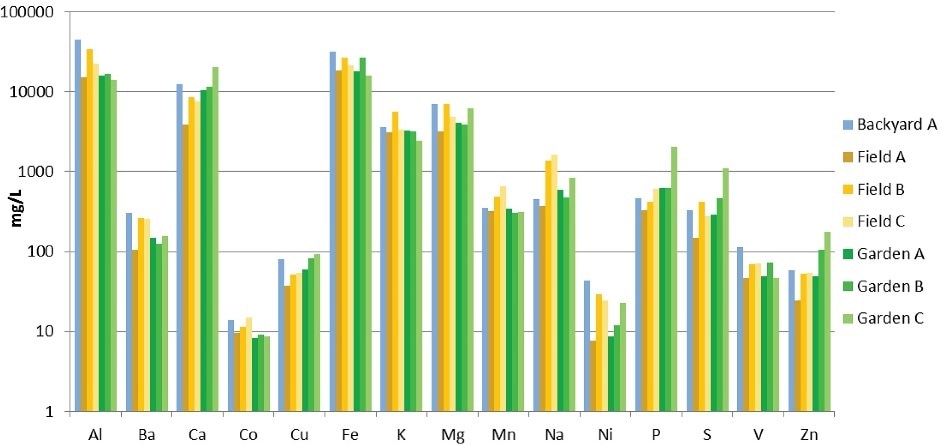
Figure 1. Results from analyses of soil samples (logarithmic scale).
Every element could be measured in a single analysis at a single sample dilution since with a large dynamic range and the Avio 200 ICP-OES’s Dual View capability, it was unnecessary to make multiple sample dilutions for elements at high and low relative concentrations.
This led to increased productivity and higher sample output. Calibration curves covering the appropriate concentration range for each element, as well as the use of calibration check solutions, sample spikes, and ICVs ensured accurate analysis.
All samples were spiked before digestion with all elements at the levels shown in Table 8 in order to assess any remaining matrix effects from the various soil samples after digestion. The resulting spike recoveries are illustrated in Figure 2 and demonstrate that all recoveries are within 10% of their true values.
Effective digestion through the Titan MPS system removed the requirement for per-sample matrix matching or the use of a method of standard addition for accurate analysis. The spike concentrations were selected to attempt to match or approach the expected concentration of the elemental concentration in the samples. This ensures that the spike signal is meaningful in relation to the sample signal for the purposes of analytical assessment.
Table 8. Pre-Digestion Spike Levels (all units in mg/L)
Element |
Al |
Ba |
Ca |
Co |
Cu |
Fe |
K |
Mg |
Mn |
Na |
Ni |
P |
S |
V |
Zn |
Conc. |
5000 |
500 |
5000 |
50 |
100 |
5000 |
5000 |
5000 |
500 |
500 |
50 |
500 |
400 |
50 |
50 |
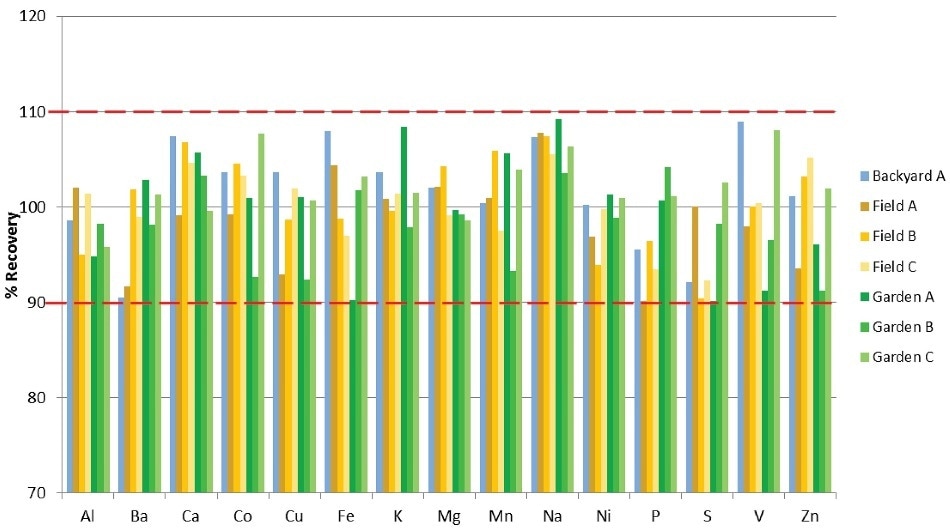
Figure 2. Spike recoveries in soil samples (% Recovery).
The analytical results and spike recoveries for the samples, as well as the confirmation of method accuracy in the measurement of CRMs, demonstrate an efficient method for the preparation and analysis of soil samples using a Titan MPS digestion system for sample preparation along with an Avio 200 ICP-OES for final sample analysis.
Conclusion
This study shows how the Avio 200 ICP-OES can effectively and reliably analyze various soil samples for several elements over a wide concentration range. With its extended capabilities, the Avio 200 ICP-OES provides greater multi-element sample output when compared to Flame AA, while permitting analysis of elements which are typically a challenge for Flame AA (such as sulfur and phosphorus).
The Titan MPS microwave digestion system simplified sample preparation while increasing the output and productivity for the laboratory in comparison with hot block or hot plate digestions. The ability to fully digest the samples, whenever needed, is a capability unmatched by open-vessel digestion.
The combined use of the Titan MPS system for sample preparation and the Avio 200 ICP-OES for analysis is ideal for simple, quick, and accurate analyses of micronutrients in soil.
Consumables Used
Avio 200 ICP-OES |
Component |
Part Number |
Red/Red PVC Pump Tubing (drain) |
09908585 |
Black/Black PVC Pump Tubing (sample) |
09908587 |
Orange/Green PVC Pump Tubing (internal standard) |
N0777110 |
Autosampler Tubes |
B0193233 (15 mL)
B0193234 (50 mL) |
Instrument Calibration Standard 2 |
N9301721 (125 mL) |
Instrument Calibration Verification Standard |
N9303953 (125 mL) |
Pure-Grade Phosphorus Standard (10,000 mg/L) |
N9303788 (125 mL)
N9300139 (500 mL) |
Pure-Grade Sulfur Standard (1000 mg/L) |
N9303796 (125 mL)
N9300154 (500 mL) |
Pure-Grade Yttrium Standard (10,000 mg/L) |
N9303810 (125 mL)
N9300167 (500 mL) |
Titan MPS Digestion System |
Component |
Part Number |
Consumables Kit for Standard 75 mL Digestion Vessels |
N3132000 |
Rupture Disks for Standard 75 mL Digestion Vessels (25 pieces) |
N3132001 |
Pressure Seal for Standard 75 mL Digestion Vessels (10 pieces) |
N3132002 |
End cap Plug for Gas Containment Manifold |
N3134004 |
Single Lip Seal Forming Tool for Standard 75 mL Digestion Vessels |
N3132015 |
8-Position Lip Seal Forming Tool for Standard 75 mL Digestion Vessels |
N3132014 |
Reference
1. Spivey, Nick, “Analysis of Micronutrients in Fruit Juice Using FAST Flame Sample Automation for Increased Sample Throughput”, Application Note, PerkinElmer, 2015.
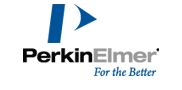
This information has been sourced, reviewed and adapted from materials provided by PerkinElmer Inc.
For more information on this source, please visit PerkinElmer Inc.