SBFSEM is an automated technique used to acquire serial images in an SEM for 3D reconstruction, developed by Denk and Horstman1 and marketed by Gatan as the 3View® system.
The resultant huge volumetric datasets can be quantitatively evaluated at a high lateral and axial resolution, similar to the analysis of mitochondrial networks in mouse pancreatic islets of Langerhans as demonstrated by Leapman and team.2
SBFSEM also has an excellent potential in materials science applications to produce 3D pictures of large materials and interfaces with nanometer resolution through large sample volumes.3
Datasets can include hundreds or even thousands of images and, hence, the loss of image quality induced by charging and contamination can adversely affect an experiment. It is important to clean and frequently replace the BSE backscatter electron detector due to deteriorating contrast from hydrocarbon contamination.
Several electron microscopists use remote plasma cleaning with the Evactron® plasma cleaner to obtain the best possible pictures from their instruments by either removing or reducing contamination.
Hydrocarbon compounds are oxidized by the oxygen radicals generated in the plasma, and this yields species, like H2O, CO and CO2, which are eliminated by the vacuum system.
XEI Scientific performs many analyses that demonstrate that the Evactron® plasma cleaner can efficiently remove hydrocarbons, and this was determined with quantifiable outcomes using formerly contaminated quartz crystal microbalances to find out the cleaning rates.4
The new study describes the advantages of plasma cleaning for the improved generation of 3View datasets and the cleaning of backscatter detectors.
Theory and Background
A typical SBFSEM system includes a SEM, a diamond-knife microtome placed on the inner wall of the chamber door of the microscope and hardware and software to control the acquisition procedure (Denk and Horstmann, 2004).
A resin-embedded sample is cut and imaged thousands of times to create serial images without user intervention. Shown in Figure 1a is an example of a typical laboratory configuration with the specialized 3View stage on the FEI Quanta 250 installed at the Institute of Biotechnology of the University of Helsinki.
Figure 1b illustrates the close-up view of the 3View microtome, which contains the illuminated sample holder on the right and the clear position of the knife on the left.
The Evactron® EP plasma cleaner (refer to Figure 1c) is compact and, hence, can be accommodated at different positions on SEM chambers depending on the availability of a suitable port, with the caveat that the port furthest away from the vacuum outlet is the best position.
Figure 1d shows an example of a hydrocarbon-contaminated backscatter detector that exhibited poor contrast and a decreased signal-to-noise (SNR).
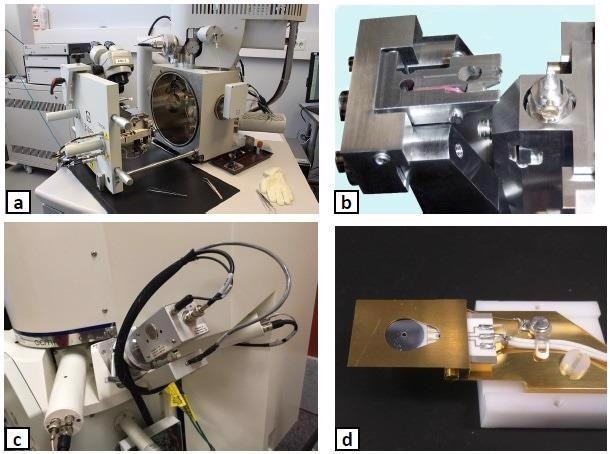
Figure 1. The hardware utilized for SBFSEM experiments: (a) a typical SBFSEM system, (b) details of the Gatan 3View microtome, (c) the Evactron® EP plasma cleaner mounted on the Zeiss Sigma SEM chamber, (d) a contaminated backscatter detector. Image Credit: Evactron (XEI Scientific)
Materials and Methods
The experiment was performed using a Sigma VP SEM from Carl Zeiss Inc. and equipped with a 3View SBFSEM System from Gatan Inc., and also an Evactron® EP decontaminator from XEI Scientific (refer to Figure 1c).
A resin-embedded mouse heart muscle sample was initially imaged at 1–3 kV, 2,000x magnification. The sample was subsequently irradiated for 10 minutes at 30 kV and then imaged to check for the formation of contaminated scanning rectangles on the machined aluminum SBFSEM stub and the loss of contrast on colloidal silver areas.
With the help of Gatan GMS 3.2 software, histograms of image contrast on colloidal silver paint regions and parts of Helicobacter pylori on Caco-2 cells both before and after cleaning were produced.
To remove hydrocarbon contamination from the specimen, chamber walls and the backscatter detector, a cleaning recipe of 5 minutes at 20 W and 2 minutes of X5 was employed.
Results
Figure 2 shows contamination artifacts (a, b) on the aluminum SBFSEM stub that go undetected after a single recipe of plasma cleaning (c, d).
Figure 3 shows a comparison of neighboring regions of colloidal silver dag, demonstrating a 14% increase in BSE contrast after one plasma cleaning cycle. The measurements were repeated three times to validate the result consistency.
The block face of embedded mouse heart tissue cleaned with a single recipe of plasma cleaning also showed an increase in BSE contrast (Figure 4).
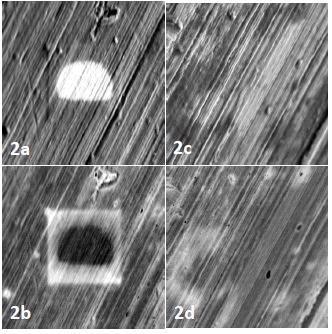
Figure 2. Contamination artifacts on the surface of the aluminum stub (a,b) are absent after plasma cleaning (c,d). Image Credit: Evactron (XEI Scientific)
To make sure that the backscattered electrons are more responsive to a contamination layer on the detector, a low accelerating voltage of 1.2 kV was applied. On applying lower accelerating voltages, the sample surfaces undergo less charging and beam damage.
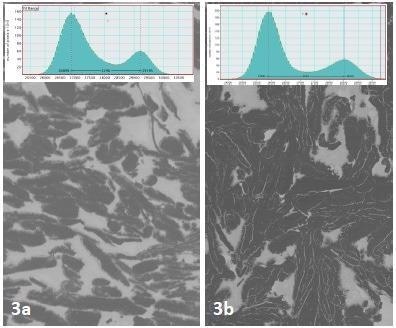
Figure 3. A 14% increase in contrast was seen after plasma cleaning a region of colloidal silver dag surrounding the SBFSEM specimen. Image Credit: Evactron (XEI Scientific)
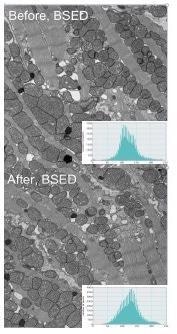
Figure 4. The block face of mouse heart tissue was scanned 20x and imaged before and after plasma cleaning. Image Credit: Evactron (XEI Scientific)
The results satisfactorily correlate with the data published in Joubert5 shown in Figure 5. In this example, the analysis of contrast levels in both pictures of resin-embedded cells quantified a 15% increase after three cycles of cleaning with 6 minutes for every cycle.
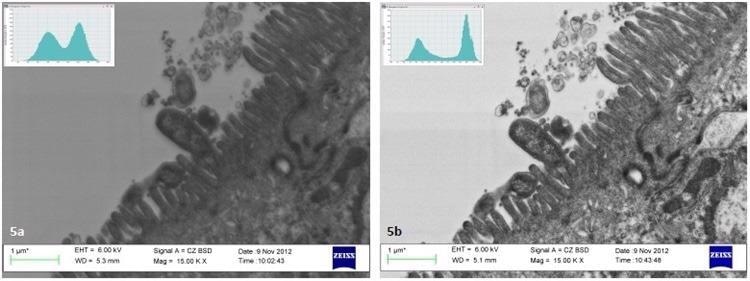
Figure 5. Analysis of images in Joubert5 indicates a 15% increase in contrast of thin sections of Helicobacter pylori on Caco-2 cells after plasma cleaning. Image Credit: Evactron (XEI Scientific)
These types of cleaning procedures help achieve precise 3D modeling and comparative morphometric studies of SBFSEM datasets by improving SNR and image contrast and eliminating imaging artifacts and charging.
In addition to extending the lifetime of backscatter detectors, the duration of the pump downtime is related to hydrocarbon contamination levels in SEMs and FIBs. Therefore, this time can potentially be used to indicate the cleanliness of the vacuum system.
The following data in Figure 6 demonstrates that the Evactron® plasma cleaners not only reduce the pump down time of the FIBs and SEMs but also reduce hydrocarbon contamination, and thus help boost the sample processing throughput without impacting the quality of the examination.
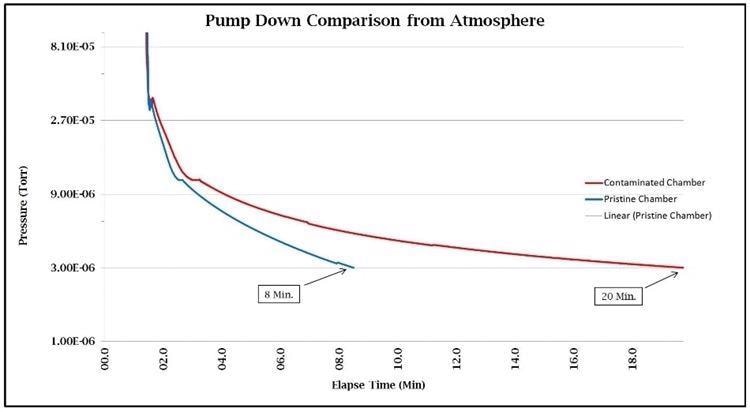
Figure 6. Image Credit: Evactron (XEI Scientific)
Oxygen radicals are formed in remote or downstream plasma cleaning and fill the vacuum chamber in the form of excited plasma. The atmospheric air is utilized as the standard process gas in Evactron® plasma cleaning but alternate gasses can be used.
The excited metastable nitrogen molecules show a flowing UV afterglow at low pressures, with a typical violet/pink color. The concentration of neutral radicals in the plasma flowing UV afterglow is a function of the rate of production in the plasma and the rate of loss in the neutral afterglow.
Conclusions
During SBFSEM experiments, the inclusion of plasma cleaning as a regular operation can improve image quality, decrease contamination artifacts and charging, expedite pump-down and sustain a clean vacuum system at the time of extended data collection.
The growing demand for extended operation in SEMs makes it significant to maintain clean conditions in the vacuum chamber. It is crucial that SBFSEM systems work around the clock and are ideally preserved in uncontaminated conditions with uncompromised quality of images.
When bulk blockface resin-embedded samples are imaged recurrently, hydrocarbons are discharged into the vacuum chamber, leading to reduced efficiency of the backscatter detector.
For instance, to ensure the ideal conditions for quick imaging, the Zeiss MultiSEM 505 is integrated with a pair of plasma cleaners to eliminate adventitious hydrocarbons from the main chamber and the load lock.
Today’s generation of Evactron® Turbo Plasma™ De-Contaminators uses a gentle, down-stream plasma afterglow procedure to remove hydrocarbon contamination from various other analytical tools, including SEMs and FIBs, while the Evactron® cleaning becomes faster and spreads all through the chamber at turbopump pressures.
This is due to the extended mean-free paths that lead to less recombination of oxygen radicals in the needed three-body collisions and reduced scattering to the walls of the chamber.
In most cases, brief plasma cleaning cycles are sufficient to remove contamination and significantly reduce pump down time, allowing high throughput of sample analysis and processing.
The Evactron® series of plasma cleaners offer robust, fast and effective cleaning across a wide range of pressures, enabling high-quality, artifact-free images and better efficiency of sample analysis. Optimal detector performance can be maintained if plasma cleaning is included in regular maintenance procedures.
References
- Denk, W. and H. Horstmann (2004) PLoS Biol. 2, 1900.
- Leapman, R. et al., (2016) Microscopy and Microanalysis 22 (Suppl. 3), 1104.
- Hashimoto, T. et al., (2016) Ultramicroscopy 163, 6.
- Vane, R. and E. Kosmowska (2016) Microscopy and Microanalysis 22 (Suppl. 3), 46.
- Joubert, L. M. (2013) Microscopy and Analysis May Issue, 15.
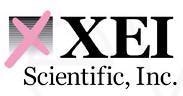
This information has been sourced, reviewed and adapted from materials provided by XEI Scientific.
For more information on this source, please visit XEI Scientific.