Within several industries, including pharma, cosmetics, inks, coatings, chemicals, food, and more, there are numerous ways to make nanoparticles. A real-time monitoring tool is lacking in commonly used "bottom-up" methods for producing nanoparticles, such as those that involve synthesizing, crystallizing, or precipitating them. Although certain particle size monitoring techniques are available for larger above-micron size particles, the submicron region is still difficult to monitor in real time. There is a broad desire to monitor the first phases of particle formation as early as feasible since the kinetics of "bottom-up" techniques are crucial for understanding and controlling these processes.
Spatially Resolved Dynamic Light Scattering (SR-DLS), a relatively new technology for inline, online and at-line nanoparticle size monitoring, was developed and introduced by InProcess-LSP in 2019. SR-DLS is capable of measuring nanoparticle size in real-time in the submicron region in flowing and undiluted nanosuspensions. In addition, high measurement frequencies of 5 to 10 seconds allow monitoring of relatively fast-changing processes as well, and provide a full ‘picture’ of the particle growth process of interest.
Technical Background: Spatially Resolved Dynamic Light Scattering is based on low coherence interferometry of backscattered broadband light (multiple wavelengths instead of a single wavelength laser). Similar to conventional DLS, intensity fluctuations due to the Brownian motion of nanoparticles are recorded and translated to diffusion rate and particle size. In addition to conventional DLS intensity fluctuations are obtained simultaneously as a function of depth in the sample up to about 3mm. Depth-resolved data enable the effective and automatic filtering of single and multiple scattered lights which results in a significantly wider accessible turbidity range compared to conventional DLS. As a result, many nanosuspensions up to relatively high concentrations or turbidity can be measured without dilution. The spatially resolved data also allows for measuring and correcting for flow. Additional movement of particles due to flow is filtered out to obtain the Brownian motion component only for particle size characterization. Measurements are performed non-invasively in samples as such or directly in the process through any glass interface such as a vial, a flow cell or a specific flask or container type.
Crystallization
The initial stage of crystallization includes nucleation which relates to the transition from an (oversaturated) liquid phase to the solid phase. After nucleation, particles start to grow and new particles will be formed in parallel by ongoing nucleation. As a result of the increasing solid concentration the driving force to nucleate due to supersaturation will decrease and particle growth will become the dominant mechanism. In Figure 1 the particle size distributions measured by SR-DLS are shown for an ibuprofen crystallization process in a glass vial (based on the addition of an anti-solvent).
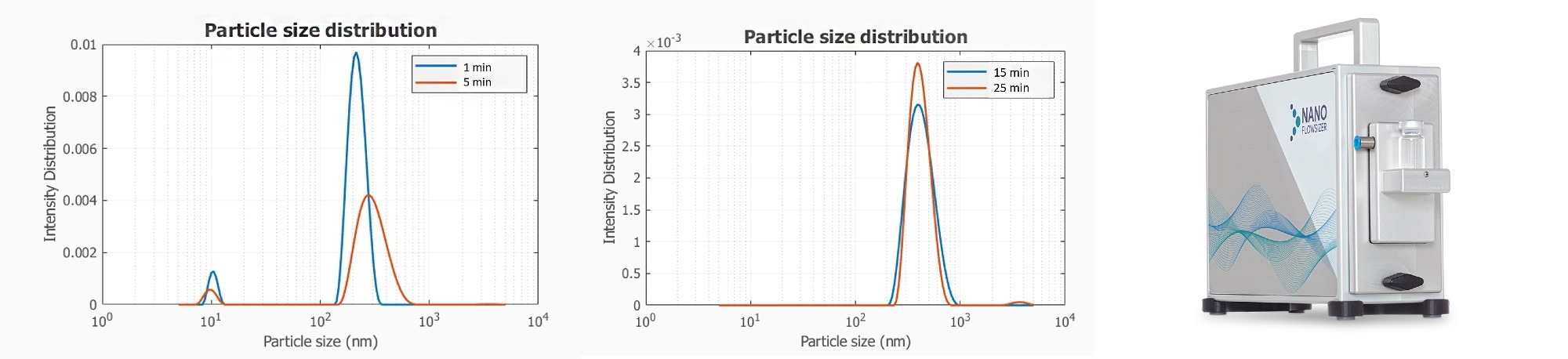
Figure 1. Real-time particle size distribution information at different time points, during the antisolvent crystallization of Ibuprofen. Image Credit: InProcess-LSP
After 1 and 5 minutes the PSD fit shows a small fraction of around 10 nm particles most likely corresponding to the formation of new particles in the nucleation stage. The fraction of small particles disappears in time, and particles of a few 100 nm start growing in time.
Additional studies with ibuprofen in 2-propanol were executed to monitor the effect of the anti-solvent (in this case water) ratio and the presence of surfactant (tween 80). In Figure 2 some examples of the monitored crystallization process in real time are shown. The real-time data shows a direct effect of different solvent/anti-solvent ratios on particle size (the more anti-solvent, the smaller the particles). The effect of the surfactant is also significant, particle size increases much faster without surfactant which is most likely due to the formation of aggregates.
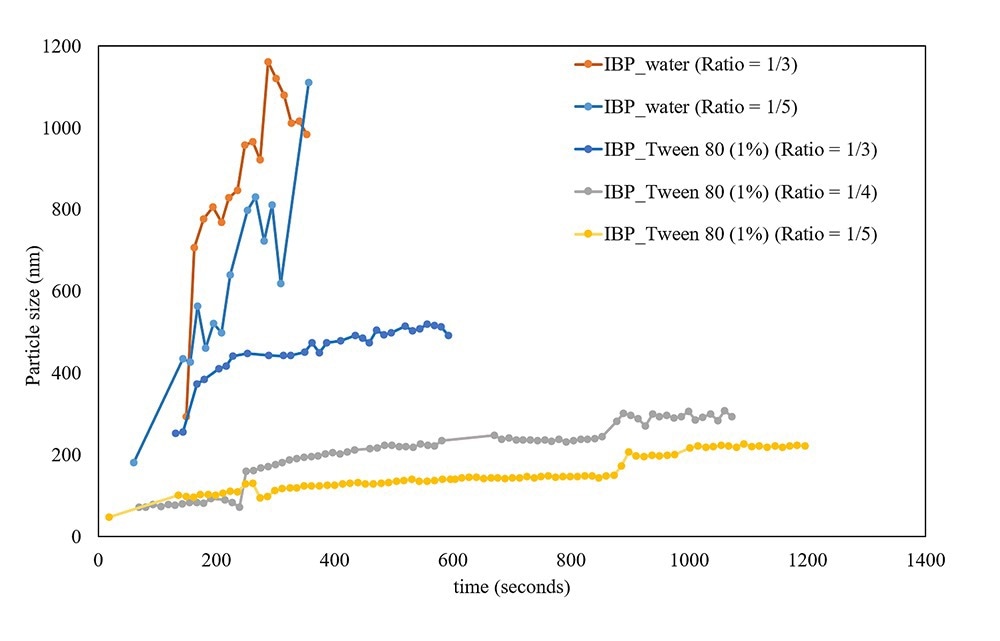
Figure 2. Real-time information about the growth of particles during the antisolvent crystallization of ibuprofen from a 2-propanol solution using different amounts of water (with or without Tween 80) as anti-solvent. Image Credit: InProcess-LSP
Shown data were all recorded in a static glass vial mode. Since no sample handling is required and measurements are performed non-invasively through the glass wall of the vial, this type of crystallization study can be executed conveniently at low volumes in a rather simple manner.
Synthesis
Particle growth of titanium dioxide particles was monitored online in real time by SR-DLS. A different setup compared to the crystallization example was applied to show the applicability of SR-DLS to measure flowing suspensions as well. An online loop connected to a micro-flowcell sampling module of a NanoFlowSizer with a small peristaltic pump circulated the reaction mixture continuously, and particle size measurements were recorded every 10 seconds. Several experiments were recorded in real time for which the amount of added KCl in the reaction mixture was varied to obtain differently sized particles.
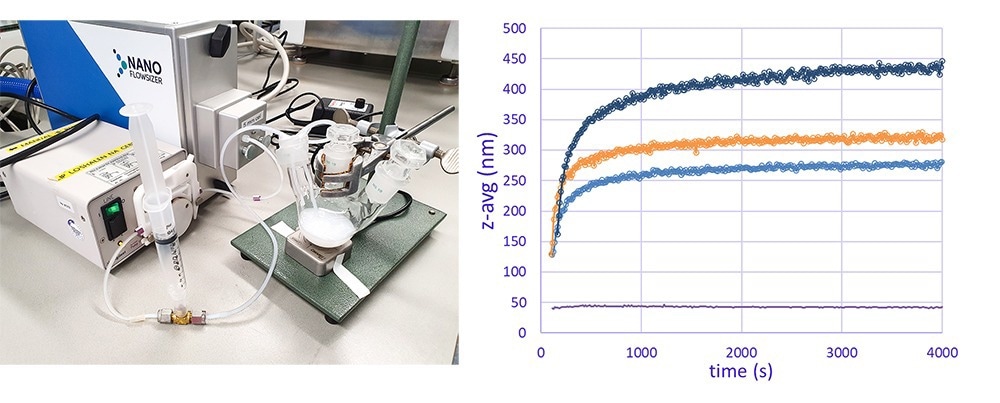
Figure 3. A) Real-time monitoring of nanoparticle size during titanium oxide synthesis by SR-DLS via online loop; B) Online real-time particle size information of synthesized TiO2 particles for different KCl concentrations. Image Credit: InProcess-LSP
The SR-DLS technology can measure over a wide turbidity range which allows continuous and real-time measurements up to relatively high concentrations of even highly scattering materials (like titanium dioxide).
Particle Growth Versus Aggregation
In the previous examples, SR-DLS showed to be suitable for real-time monitoring of particle growth processes. However, particle size data as such does not distinguish between ‘real’ particle growth and particle aggregation. Besides particle size information, backscatter intensity as recorded by SR-DLS is obtained simultaneously for each particle size measurement and is directly related to the concentration (in principle turbidity) of the suspension. In case of particle growth the concentration increases, while the concentration remains unchanged in case of aggregation. Both mechanisms may be identified based on backscatter intensity data.
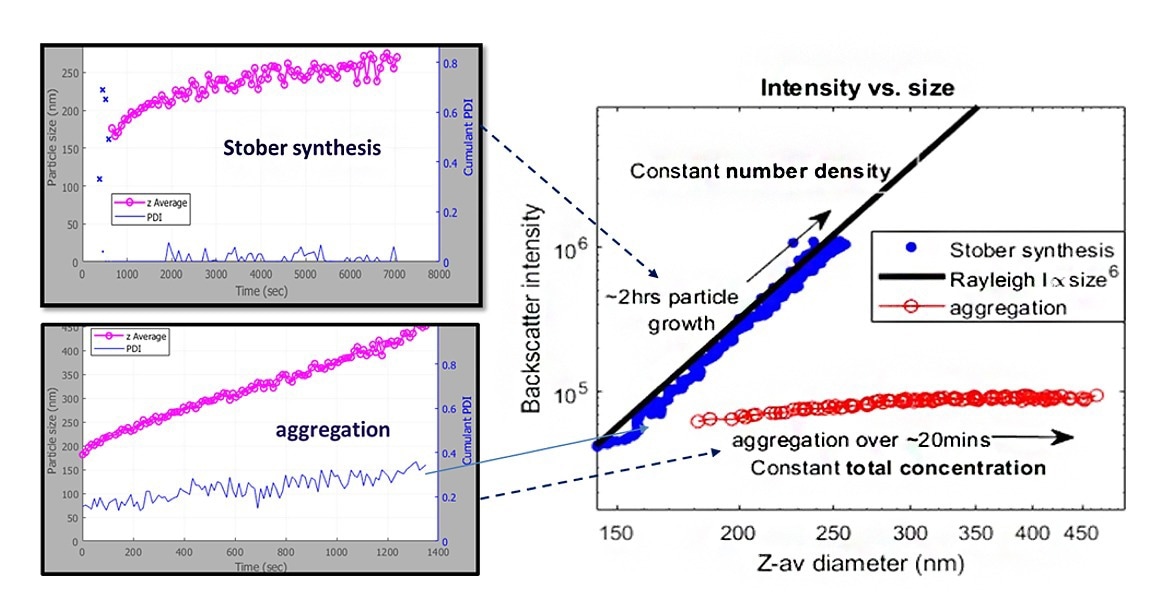
Figure 4. SR-DLS particle size and backscatter intensity of silica particle growth (Stöber) and aggregation. Image Credit: InProcess-LSP
In Figure 4 two processes involving the formation of silica particles show increasing particle size over time. One of the processes is based on actual particle growth (Stöber synthesis) and one of the processes is based on aggregation only by adding salt to 100nm silica particles. For a situation where particles only grow (with constant number density), the backscatter intensity associated with particle growth (Stöber) shows a close correlation with the expected increase in turbidity (based on Raleigh scattering). The obtained backscatter intensity of the silica suspension with added salt shows a nearly constant backscatter intensity indicating no significant change in concentration and therefore indicating aggregation.
Conclusions
Spatially Resolved Dynamic Light Scattering offers new opportunities to monitor the growth of nanoparticles in real time. The fast data acquisition allows monitoring of particle size and particle size distribution every 5 to 10 seconds. Due to the ability to measure over a wide turbidity range in static and flowing suspensions, different measurement configurations can be applied to monitor particle size formation during crystallization or synthesis. The combination of the simultaneously obtained backscatter intensity with particle size information allows distinguishing between particle growth and particle aggregation.
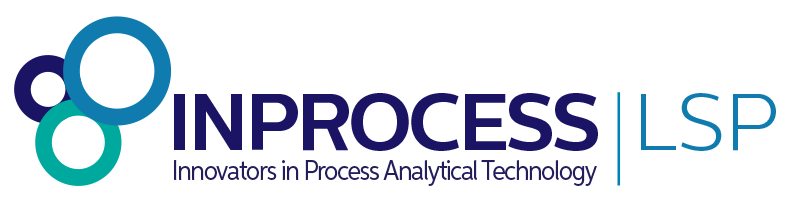
This information has been sourced, reviewed and adapted from materials provided by InProcess-LSP.
For more information on this source, please visit InProcess-LSP.