The use of renewable energies has gained momentum in recent years in order to combat climate change. It is also one of the top commercial priorities as industries and individuals work together to reduce their energy consumption.
Today, the popularity of so-called ‘clean energy’ remains limited by a number of factors, including the fact that power fluctuates across a variety of time frames – a particularly challenging issue to combat.
In addition, the transition to zero-emission sources of electricity is hindered by the limited efficiency of available lithium batteries.1
Alternatively, by employing a chemical reaction to convert an energy source into electricity, fuel cells avoid the time-dependent generation, transport and storage of electrical energy issues.
Proton exchange membrane fuel cells (PEMFCs), in particular, are viewed as highly promising sources of renewable power for transportation and other mobile applications.2
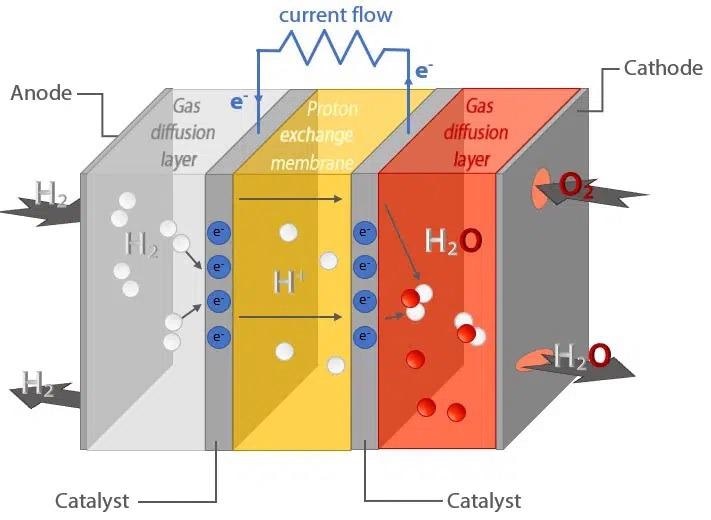
Figure 1. Schematic representation of a proton exchange membrane fuel cell. Two chemical reactions take place at the anode ( 2H2 -> 4H+ + 4e–) and cathode (O2 + 4H+ + 4e– -> 2H2O) consequently generating a current flow. Image Credit: Xenocs
The Degradation Processes of Carbon-Supported Platinum Electrocatalysts
Carbon-supported platinum or platinum alloy nanoparticles are currently the most exploited electrocatalysts for PEMFCs in order to catalyze oxygen reduction and hydrogen oxidation reactions.
However, large-scale commercialization is hindered by the high cost and scarcity of Pt. A significant amount of research nowadays concentrates on understanding the degradation mechanisms with the ultimate goal of increasing the catalytic activity and stability to make this type of fuel cell competitive for practical applications.
In order to reduce the testing time during experiments, accelerated stress tests (ASTs) are routinely employed. Two degradation paths have been distinguished as a function of applied potential.3
- For operation potentials between ≈ 6 and ≈ 1 V: at the expense of smaller ones, electrochemical Ostwald ripening will favor the formation of larger Pt nanoparticles.
- For excess cathode potentials of ≈ 5 V: the carbon support’s corrosion will bring about the detachment and ultimately the coalescence of Pt nanoparticles.
An essential part of the research aiming to understand the degradation of electrocatalyst materials is the study of particle size distribution at the nanoscale.
Small-angle X-Ray scattering (SAXS) is a powerful technique that can offer valuable information for this purpose, specifically about the morphology of the catalyst (size, shape, and size distribution of nanoparticles).
Either ex-situ measurements performed on a large number of samples or through more suitable in-situ or operando measurement can be used to infer the time-resolved evolution of particle size distribution during the catalyst degradation cycle.
Typically, operando SAXS experiments are performed by use of a synchrotron X-Ray source as a result of their intense beams and straightforward background subtraction of the carbon support.3
Typically, the background is measured ex-situ on a platinum-free carbon electrode subjected to the same degradation process as the studied one for measurements performed on laboratory sources.
Often, this requires a secondary separate experiment which can bring about further errors in the normalization process if the measuring conditions are not the same.4
Advances in data acquisition offer the possibility to record high-quality operando SAXS data of catalyst degradation with a laboratory X-Ray source.
A research group from the University of Copenhagen and the University of Bern has recently proposed a new design for the working electrode.5
This new design allows the recording of the operando background data on a section of the cell which is catalyst-free, which undergoes the same electrochemical treatment as the catalyst film.
Within their proof-of-concept study, the research team revealed that proper background subtraction can be obtained for operando SAXS measurements performed on laboratory X-Ray sources as a part of a single experiment.
A comparison is made between probability density functions obtained using background data gathered before and after the accelerated stress tests (i.e., with the newly-designed working cell) and data recorded on a pristine electrolyte-free sample measured ex-situ (see the figure below).
It was primarily observed that a better agreement with the pristine sample was achieved with normalization to the background scans recorded after the electrochemical test (Figure 2b) as compared to normalization to background scans recorded before the electrochemical test (Figure 2a).
Moreover, two transformations become evident when analyzing the particle size distribution as a function of AST steps and using a model comprising two distinguishable size particle populations, as evidenced in Figure 2 (b):
- While the size of large particles remains constant, the size of particles belonging to the small particles population increases continuously.
- Concomitant with an increase of the probability density of large particles, the probability density of small particles decreases.
Therefore, it is possible to conclude that operando SAXS measurements reveal that the degradation of the Pt/C fuel cell consists of a continuous dissolution of Pt step when subjected to ASTs, followed by Oswald ripening, in contrast with the alternative scenario of particle coalescence.
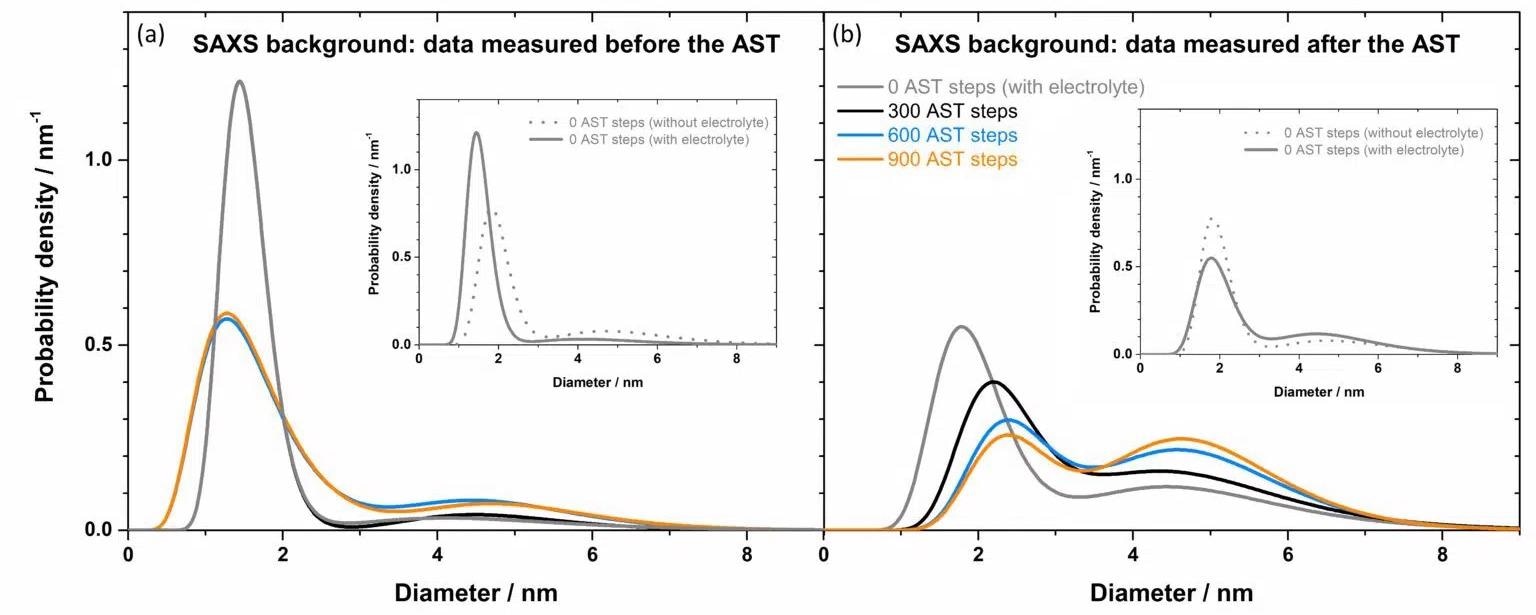
Figure 2. Probability density as a function of particle diameter, as obtained from SAXS measurements, recorded at various instants during the AST cycle. (a) Data normalized to background scans recorded before the AST cycle. (b) Data normalized to background scans recorded after the AST cycle. The inset of each figure depicts the comparison between the sample before applying the AST cycle (and normalized to its respective background scan) and a pristine ex situ sample (measured without electrolyte). Courtesy of Johanna Schröder and Jacob J. K. Kirkensgaard. Image Credit: Xenocs
The possibility of acquiring high-quality operando SAXS data of catalyst degradation with a laboratory X-Ray source and a modified electrochemical cell is demonstrated by this proof-of-concept study.
The advancements have opened the door to in-house measurements in background subtraction, which, in turn, offer more flexibility for reproducibility studies and experiment optimization as compared to synchrotron beamtimes.
Simultaneously, preliminary SAXS data acquired in a laboratory setting can be used to design more efficient synchrotron experiments.
This research first appeared in the following article [5] Schröder, Johanna, Jonathan Quinson, Jacob J. K. Kirkensgaard, and Matthias Arenz. “Operando SAXS study of a Pt/C fuel cell catalyst with an X-ray laboratory source.” Journal of Physics D: Applied Physics 54, no. 29 (2021): 294004.
References
- Mauger, Alain, and C. M. Julien. “Critical review on lithium-ion batteries: are they safe? Sustainable?” Ionics 23, no. 8 (2017): 1933-1947.
- Chandan, Amrit, Mariska Hattenberger, Ahmad El-Kharouf, Shangfeng Du, Aman Dhir, Valerie Self, Bruno G. Pollet, Andrew Ingram, and Waldemar Bujalski. “High temperature (HT) polymer electrolyte membrane fuel cells (PEMFC)–A review.” Journal of Power Sources 231 (2013): 264-278.
- Povia, Mauro, Juan Herranz, Tobias Binninger, Maarten Nachtegaal, Ana Diaz, Joachim Kohlbrecher, Daniel F. Abbott, Bae-Jung Kim, and Thomas J. Schmidt. “Combining SAXS and XAS to study the operando degradation of carbon-supported Pt-nanoparticle fuel cell catalysts.” ACS Catalysis 8, no. 8 (2018): 7000-7015.
- Tillier, Jérémy, Tobias Binninger, Marios Garganourakis, Alexandra Patru, Emiliana Fabbri, Thomas J. Schmidt, and Olha Sereda. “Electrochemical Flow-Cell Setup for In Situ X-ray Investigations.” Journal of The Electrochemical Society 163, no. 10 (2016): H913.
- Schröder, Johanna, Jonathan Quinson, Jacob J. K. Kirkensgaard, and Matthias Arenz. “Operando SAXS study of a Pt/C fuel cell catalyst with an X-ray laboratory source.” Journal of Physics D: Applied Physics 54, no. 29 (2021): 294004.
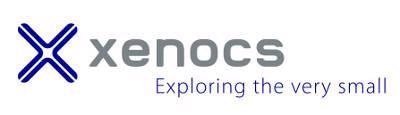
This information has been sourced, reviewed and adapted from materials provided by Xenocs.
For more information on this source, please visit Xenocs.