This article discusses the application of transmission electron microscopy (TEM) to analyze metal nanoparticles.
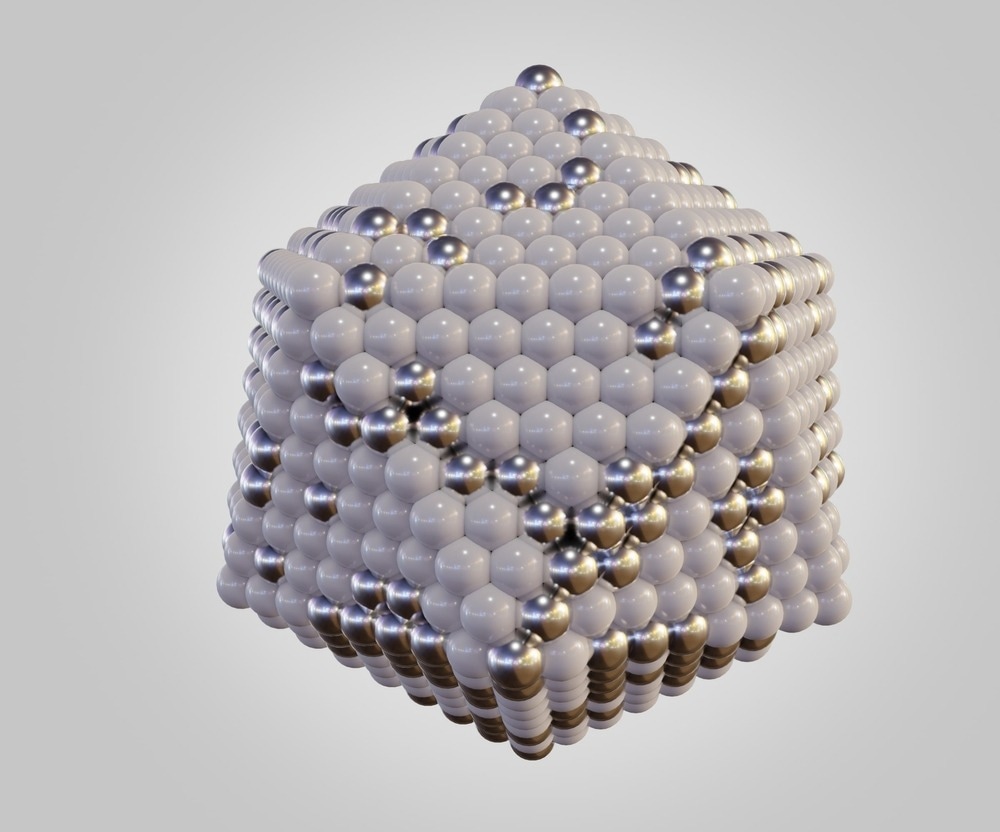
Image Credit: Love Employee/Shutterstock.com
Importance of TEM for Metal Nanoparticles
Nanometer-scale analytical and structural characterization of different materials has gained significant attention in recent years. TEM is considered suitable for nanometer scale characterization as parameters such as phase type, chemical composition, crystallographic details, morphological information, lattice type, grain size, and particle size can be obtained from transmission electron micrographs.
Electron diffraction patterns of nanomaterials are used to obtain quantitative information, including crystal defects in the lattice structure, orientation relationship, size, and phase identification. Thus, several studies on metal nanoparticles have been performed using TEM.
TEM observations have revealed the structural and configurational fluctuations and the real space structure of the nanoparticles. Moreover, TEM observation has also provided information about the behavior of nanoparticles in the electron beam.
Application of TEM on Metal Nanoparticles
In a study published in the journal Micron, researchers performed structural observation of molybdenum, palladium, and indium nanoparticles deposited on silicon (110) substrates using an ultrahigh vacuum field emission TEM (UHV-FE-TEM).
The three metals were selected based on their melting point to evaluate their behavior dependence on temperature. The melting points of molybdenum, palladium, and indium are 2893 K, 1825 K, and 430 K, respectively.
A UHV-FE-TEM microscope with 200 kV accelerating voltage, 0.1 nm lattice resolution, and 0.21 nm point resolution at Scherzer defocus was used for this study. The microscope was also equipped with a tungsten/zirconium oxide Schottky-type thermal field emission gun (FEG) with 0.7 eV energy spread.
Moreover, two evaporators, including a thermal heating evaporator with tungsten wire for different materials and an electron beam evaporator for high melting point materials, were attached to the microscope column to perform dynamic and in situ evaporation of the samples.
Indium nanoparticles of 3-5 nm in size displayed frequent structural fluctuations under 101 A/cm2 electron beam irradiation. In comparison, indium nanoparticles of more than 10 nm in size, including decahedral multiply twinned particles (MTPs), demonstrated a slower fluctuation compared to smaller nanoparticles. Additionally, the bigger nanoparticles displayed coalescence with a weaker 100 A/cm2 beam.
Palladium nanoparticles with a size of 3-5 nm showed structural fluctuations after 10-30 s of electron beam irradiation. However, a stronger beam irradiation with 103 A/cm2 intensity resulted in the dissipation of the palladium nanoparticles due to diffusion.
No structural fluctuation was observed in molybdenum nanoparticles of 3-5 nm. However, intensive electron beam irradiation with 105 A/cm2 intensity led to the dissipation of the nanoparticles.
Studies have demonstrated that the atomic number (Z)-contrast capabilities of high angular annular darkfield-scanning TEM (HAADF-STEM) can be utilized to investigate metal nanoparticles. For instance, decahedra gold nanoparticles can be identified at the atomic scale using HAADF-STEM.
Gold-palladium bimetallic core-shell structures were successfully identified by differences in contrast in the HAADF-STEM imaging. In the imaging, the high contrast corresponded to the gold shell with atomic number 79, while the lower contrast corresponded to the palladium core with atomic number 46.
The incorporation of metal nanoparticles into biological systems in a controllable manner has remained a significant challenge. STEM imaging can become an important tool for identifying the influence and incorporation of different shapes into biological systems.
Biological systems primarily contain carbon, hydrogen, and nitrogen as the matrix. The contrast of these elements in HAADF-STEM imaging is lower than the contrast generated by metal nanoparticles.
Thus, the method can enable easy identification of embedded metallic nanostructures in biological tissue. For instance, silver nanoparticles incorporated into bacteria were identified by contrast in HAADF-STEM imaging.
Recent Studies Involving TEM Imaging of Metal Nanoparticles
TEM images of metallic nanoparticles are acquired by dispersing a large number of nanoparticles on a thin amorphous silicon nitride/amorphous carbon foil that is transparent to incident electrons.
In a TEM study, the first stage involves the selection of a target nanoparticle that can provide crucial information required to understand the mechanism underlying the material function and/or structure of the material.
However, the selection of a target nanoparticle among several nanoparticles has remained a major challenge in the data collection process while using TEM, as this is a time-consuming operation.
A similar problem also exists in the data analysis stage in which the target images must be selected from several TEM observations. These technical issues have necessitated the development of automated systems for the collection and classification/identification of metallic nanoparticles in TEM images.
Deep learning can play a significant role in automating both TEM data analysis and data collection. In a recent study published in the Journal of Magnetism and Magnetic Materials, researchers developed a TEM image analysis method using transfer learning and convolutional neural networks (CNNs) to achieve precise identification of nanoparticles.
CNNs pre-trained by transfer learning were used to analyze the TEM images of nanoparticles. Platinum nanoparticles that generate strong contrast in TEM images when supported on an electron-transparent titanium dioxide foil were used as a test subject. TEM images of platinum nanoparticles dispersed on a thin titanium dioxide crystal foil were classified using CNNs.
Although the number of learning data was several orders of magnitude smaller than the quantities typically utilized in conventional CNN analyses, the CNN model in this study was able to classify images with 94% accuracy after the convolutional layers (CLs) were pre-trained by transfer learning and fine-tuning compared to 93% accuracy normally achieved by a trained electron microscopist.
Thus, the transfer learning method can be feasibly used for TEM studies of both magnetic and non-magnetic nanoparticles, as only a limited number of TEM images is required in this method for the learning dataset.
References and Further Reading
Tanaka, M., Takeguchi, M., Furuya, K. (2002). Behavior of metal nanoparticles in the electron beam. Micron, 33(5), pp. 441-446. https://www.sciencedirect.com/science/article/abs/pii/S0968432801000464
Koyama, A., Miyauchi, S., Morooka, K., Hojo, H., Einaga, H., Murakami, Y. (2021). Analysis of TEM images of metallic nanoparticles using convolutional neural networks and transfer learning. Journal of Magnetism and Magnetic Materials, 538, p. 168225. https://www.sciencedirect.com/science/article/abs/pii/S0304885321005011
Ponce, A., Mejía-Rosales, S., Yacaman, M. (2012). Scanning Transmission Electron Microscopy Methods for the Analysis of Nanoparticles. Methods in molecular biology. doi.org/10.1007/978-1-61779-953-2_37
Disclaimer: The views expressed here are those of the author expressed in their private capacity and do not necessarily represent the views of AZoM.com Limited T/A AZoNetwork the owner and operator of this website. This disclaimer forms part of the Terms and conditions of use of this website.