The advent of 2D materials has unlocked exceptional functionalities, from electronics to energy storage, that promise transformative technologies. However, significant challenges have emerged with their rising demand, hindering their widespread and sustainable adoption in a circular economy.
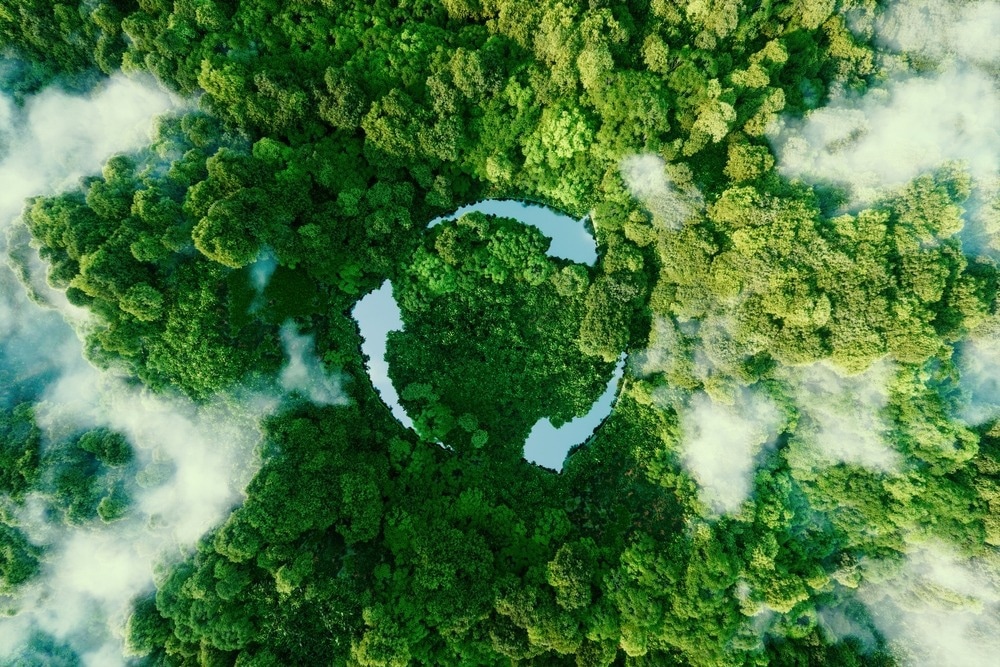
Image Credit: petrmalinak/Shutterstock.com
The production and isolation of single-layer graphene in 2004 sparked global interest in 2D materials. The unique structures and properties of these atomically thin materials arise from the hybridization and quantum confinement of their electrons when structured in layered planes rather than 3D bulk.
The portfolio of 2D materials has since grown enormously, each with tuned properties catering to different uses. Early progress focused predominantly on lab-scale proof-of-concept studies into these materials' capabilities. However, the last decade's exponential rise in publications and patents signals the transition towards commercialization and real-world implementation is well underway.
For instance, global revenues from graphene products alone could surpass $1.64 billion by 2034. However, the increasing use and development of 2D materials raises important questions regarding sustainability within a circular economy framework.
Challenges and Limitations of Conventional Production Techniques
Current large-scale manufacturing techniques rely extensively on resource-intensive and emission-heavy processes that undermine the environmental credentials of 2D materials. The most common top-down methods to produce graphene and transition metal dichalcogenides (TMDs) use exfoliation routes that require significant energy usage, utilize hazardous chemicals, produce toxic byproducts, and face issues around feedstock purity.
Popular chemical vapor deposition (CVD) synthesis needs high temperatures (around 1000°C) and suffers from scaling constraints due to the need for specific catalytic substrates for epitaxial growth. This leads to compromised crystalline quality, increased defects, metallic impurities, and potential polymer residuals altering the properties of transferred 2D material flakes.
On the other hand, liquid phase exfoliation (LPE) that exfoliates bulk graphite in solvents like N-Methylpyrrolidone (NMP) and Dimethylformamide (DMF) has demonstrated scalability but relies on non-recyclable and increasingly regulated solvents. In addition, the carbon footprint from LPE can still approach 620g CO2 equivalent per gram of graphene yield.
A major confrontation hampering the eco-responsible scaling of 2D materials is the prevailing reliance on mineral mining. With contemporary mining rates projecting the depletion of high-quality deposits within the coming decades, such finite feedstock dependency threatens output stability. Simultaneously, artificially synthesizing minerals as an alternative produces even higher carbon emissions due to the intensive heat and chemical inputs needed, undermining sustainability pursuits.
Apart from process sustainability, the quality and consistency also vary widely for conventionally produced 2D materials - especially graphene - with imprecise control on layer number, defects, and oxidation. This contributes to technology stagnation as inconsistent graphene translates to unreliable device performance.
Recyclability Roadblocks
The eventual fate of incorporated 2D fillers in commodity composites and coatings remains a sustainability dilemma, given the complexity and costs of recovering these nanomaterials after end-of-life.
The extreme durability of 2D materials, which makes them so useful in products, also hinders degradation, preventing the recovery and reuse of their constituents. As a result, generic sorting and recycling techniques fail to detach and re-exfoliate graphene or TMDs post-matrix incineration or landfilling efficiently.
Scalable solutions are needed to conserve their intrinsic value and prevent environmental release through enhanced detachment, purification and reuse protocols built into product designs.
Toxicity Apprehensions
Another rising issue is the toxicological and ecotoxicological profiling of engineered 2D materials. Preliminary biodegradation pathways have been mapped, but an in-depth understanding of how the transformation kinetics and products might affect ecological bioaccumulation, biomagnification along nutritional chains, or organismal exposure risks is lacking.
The occupational health hazards involved in nanomanufacturing and lifecycle processing of 2D materials represent an additional domain requiring risk analysis. Therefore, standardizing cytotoxicity testing methods is essential to ensure reliable safety screening.
Economic Constraints
Overcoming financial barriers is crucial for transitioning to greener synthesis pathways for 2D materials. Substituting conventional toxic reagents with bio-based alternatives or waste precursors initially carries green premiums that deter industry adoption. In addition, quality variations also continue to be a prime issue with such precursors.
Therefore, constructing robust, eco-conscious process cost models for externality mitigation is essential to sway small margins-driven decision-making and boost competitiveness.
Promising Sustainable Advancements
Recent technological breakthroughs offer optimism that most prevailing shortcomings can be effectively addressed to transition toward eco-friendly nanomanufacturing high-grade 2D materials without compromising economic viability.
A recent study published in One Earth proposed a flash joule heating (FJH) method that relies solely on electricity to dissociate waste-derived carbon sources like plastic or used tires into defect-free graphene in microseconds. FJH can produce high-quality graphene from biomass waste, significantly reducing carbon and water footprints by over 90% while offering cost-effective advantages compared to existing technologies.
Moreover, graphene can now be produced sustainably from waste methane, as demonstrated by Levidian. This innovative process, involving decarbonization to create hydrogen and graphene, supports a circular economy through local supply chains, eliminating the need for environmentally impactful and geopolitically challenging rare metal imports.
With suitable incentivization, such methods can decarbonize the industry and provide circular economic inroads to advance a low-carbon economy.
Future Outlook
The advent of 2D materials heralds a new era of material capabilities and innovation potential. However, achieving a sustainable, circular 2D materials economy remains a multifaceted grand challenge spanning everything from lab bench advances to end-of-life infrastructure overhauls to policy development.
An integrated approach is vital to promote the responsible use, circulation and governance of 2D materials for the decades ahead. With vision and collaboration, innovative circularity solutions can emerge to match the pace of 2D material development and expansion.
References and Further Reading
Jia, C., Pang, M., Lu, Y., Liu, Y., Zhuang, M., Liu, B., ... & Yang, Y. (2022). Graphene environmental footprint greatly reduced when derived from biomass waste via flash Joule heating. One Earth, 5(12), 1394-1403. https://doi.org/10.1016/j.oneear.2022.11.006
Britnell, L. (2021). Graphene lifecycle: how sustainable are emerging nanotechnologies? [Online]. Available at: https://www.mub.eps.manchester.ac.uk/graphene/2021/12/graphene-lifecycle-how-sustainable-are-emerging-nanotechnologies/
Shams, M., Mansukhani, N., Hersam, M. C., Bouchard, D., & Chowdhury, I. (2023). Environmentally sustainable implementations of two-dimensional nanomaterials. Frontiers in Chemistry, 11, 1132233. https://doi.org/10.3389/fchem.2023.1132233
Khan, K., Tareen, A. K., Aslam, M., Sagar, R. U. R., Zhang, B., Huang, W., ... & Guo, Z. (2020). Recent progress, challenges, and prospects in two-dimensional photo-catalyst materials and environmental remediation. Nano-Micro Letters, 12, 1-77. https://doi.org/10.1007/s40820-020-00504-3
Occhiuzzi, J., Politano, G. G., D’Olimpio, G., & Politano, A. (2023). The Quest for Green Solvents for the Sustainable Production of Nanosheets of Two-Dimensional (2D) Materials, a Key Issue in the Roadmap for the Ecology Transition in the Flatland. Molecules, 28(3), 1484. https://doi.org/10.3390/molecules28031484
Fojtů, M., Teo, W. Z., & Pumera, M. (2017). Environmental impact and potential health risks of 2D nanomaterials. Environmental Science: Nano, 4(8), 1617-1633. https://doi.org/10.1039/c7en00401j
Disclaimer: The views expressed here are those of the author expressed in their private capacity and do not necessarily represent the views of AZoM.com Limited T/A AZoNetwork the owner and operator of this website. This disclaimer forms part of the Terms and conditions of use of this website.