MXenes are a class of 2D materials that are defined by having a composition of Mn+1XnTx., where M is a transition metal, X is nitrogen or carbon and T is some kind of surface functional groups.1 Most MXenes are transition metal carbides and nitrides and some of the most famous examples include Ti3C2Tx, which was one of the first MXenes to be discovered.
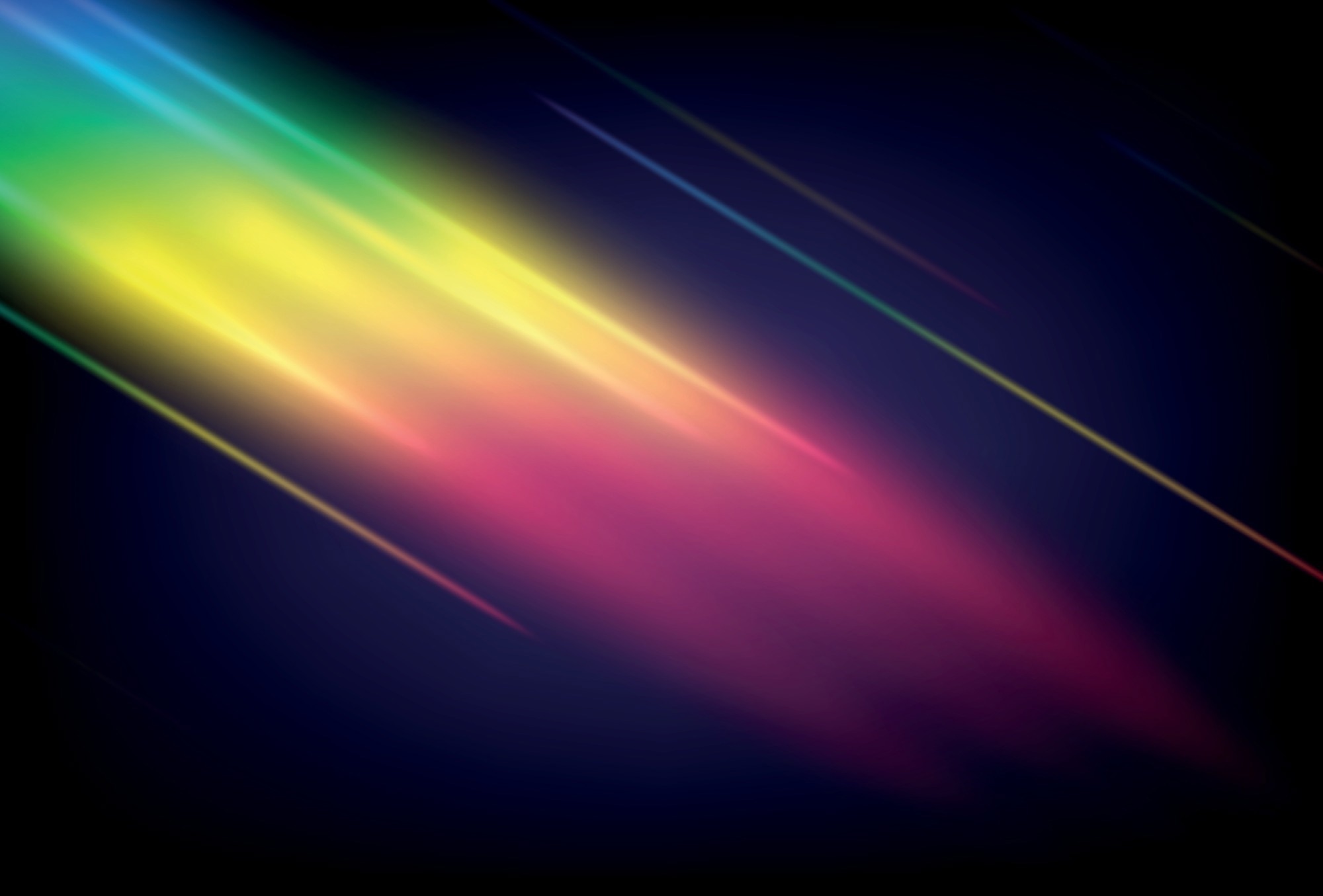
Image Credit: Apostle/Shutterstock.com
There are around 20 known MXene structures at present, and some of the main excitement around these materials comes from some of their potential applications, including as energy storage materials.2 MXenes have shown great promise in terms of theoretical gravimetric capacity for use in battery technologies as well as the ability to tune the ion penetration between layers with the chemical structure of the MXene.
Other examples of MXene applications include water purification, such as for the absorption of lead or other heavy metals,3 or desalination membranes. The ability to act as a selective ion filter is particularly useful for all these applications as the MXene can be designed to reject only the unwanted species. The high thermal and radiation stability of MXenes can also help in many ion filtration applications, including nuclear waste management.4
Structural Importance
The structural arrangement of the MXene is critical to all of the aforementioned filtration and purification applications. The electronic configuration and size of the interacting molecule or ionic species determine where and how the absorbate will interact with the MXene material.5 MXenes are often made through etching processes and have a complex multilayer structure made up of individual sheets of the 2D material.
The composition and arrangement of the individual sheets and their stacking distances influence the final properties of the MXene material. Therefore, a key part of MXene synthesis and development is the use of materials characterization techniques that can provide information on the atomic composition and structural arrangement both in the individual sheets and the inter-sheet distances.
Commonly used methods for MXene characterization include X-ray photoelectron spectroscopy, electron microscopy and Raman spectroscopy. Some characterization methods are particularly well-suited to certain stages of the MXene synthesis process, such as identifying precursors and the analysis of the flakes or thin films.5 Of all the characterization methods, Raman spectroscopy is well-suited to both elemental composition and providing information on the chemical bonding environments in the MXene families.
Raman Spectroscopy
Raman spectroscopy is a spectroscopic technique for measuring the vibrational frequencies in a target sample. The frequencies of the vibrations measured are dependent on both the specific atoms involved in the bond as well as the local chemical environment. As most molecules will give rise to a number of lines in a Raman spectrum, the overall spectral profile can be used to identify unknown chemical species as well as to analyze the strengths and nature of the individual bonds.
A Raman spectra is recorded by shining an intense light source, typically a laser, and measuring the intensity and the energy of the scattered light. The Raman shift, the frequency shift between the incident and the scattered light, corresponds to the vibrational modes in the molecule.
For MXenes, there have only been a limited number of Raman spectra published in the literature, meaning there are not extensive databases of existing spectral information for compound identification.6 However, the sensitivity of Raman spectroscopy to local chemical environments makes it a powerful tool for examining the surface terminations, layer thickness and the degree of metallic properties.6
For another classic 2D material, graphene, Raman spectroscopy has been used to provide information about the material quality, crystal orientation, strain and identify the overall material quality. Similar information should be recoverable for MXenes, with the addition of information about the electronic structure, as it is possible to excite MXenes in the band gap with the choice of the correct excitation wavelength.6
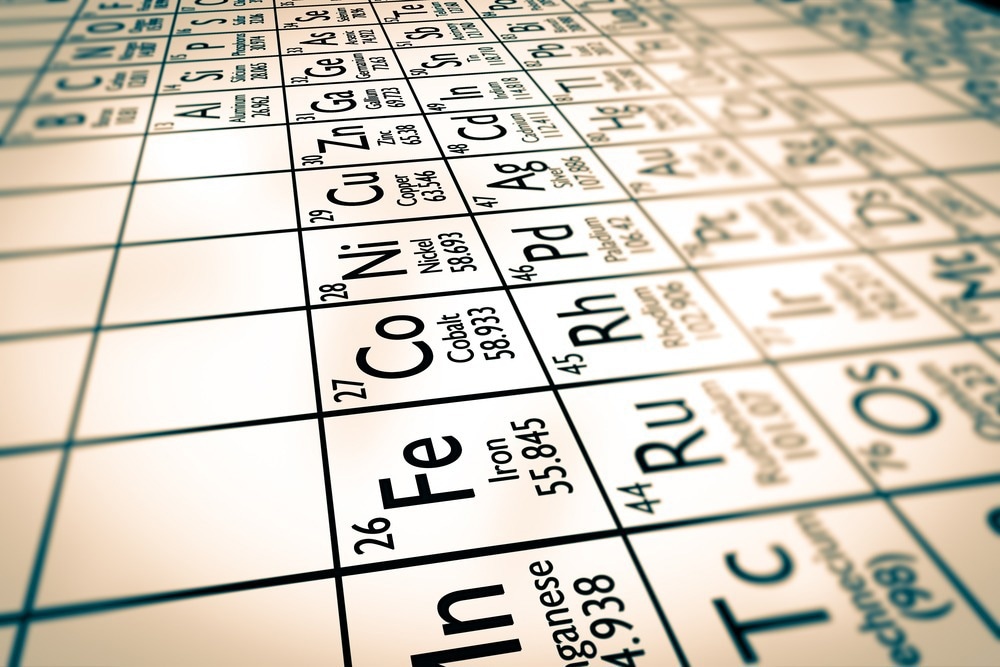
Image Credit: Antoine2K/Shutterstock.com
MXene Characterization
One of the effects observed in the Raman spectra as the individual MXene flakes are layered to form a multilayer material is that the peaks broaden due to the intermolecular interactions between the layers.5 Many of the typical terminal groups used in MXene synthesis, such as carbonyls or hydroxyl groups, have strong and distinctive Raman signatures that make it possible to identify the chemical formulae of the MXene.
Raman spectroscopy can be used for quantitative analysis with the right use of reference standards. The peak intensities are proportional both to the transition strength and the number of groups present with the same type of transition i.e. how many of a particular terminating group is present.
In comparison to techniques such as XRD, which may give very similar results for chemically dissimilar MXenes that have similar overall structures.5 Other types of Raman spectroscopy, such as polarized Raman spectroscopy, can provide additional information on the nature of the vibrations and their symmetry – such as whether they are in or out of the plane.
With the availability of more MXene reference spectra, identifying chemically unknown MXene structures will be simplified and more research can be done into recovering more complex structural information.5
References and Further Reading
Chakraborty, P., et al. (2019). 1.15 - MXene: A New Trend in 2D Materials Science. In D. L. Andrews, R. H. Lipson, & T. Nann (Eds.), Comprehensive Nanoscience and Nanotechnology (Second Edition) (Second Edition, pp. 319–330). Academic Press. doi.org/10.1016/B978-0-12-803581-8.10414-X
Anasori, B., et al. (2017). 2D metal carbides and nitrides (MXenes) for energy storage. Nature Materials Reviews, 2, p.16098. doi.org/10.1038/natrevmats.2016.98
Peng, Q., et al. (2014). Unique Lead Adsorption Behavior of Activated Hydroxyl Group in Two-Dimensional Titanium Carbide. Journal of the American Chemical Society, 136, pp.4113–4141.
Wang, L., et al. (2016). Loading Actinides in Multilayered Structures for Nuclear Waste Treatment: The First Case Study of Uranium Capture with Vanadium Carbide MXene. Applied Materials and Interfaces, 8, pp.16396–16403. doi.org/10.1021/acsami.6b02989
Shevchuk, K., et al. (2023). Raman Spectroscopy Characterization of 2D Carbide and Carbonitride MXenes. Chemistry of Materials, 35, pp.8239–8274. doi.org/10.1021/acs.chemmater.3c01742
Shekhirev, M., et al. (2021). Characterization of MXenes at every step , from their precursors to single flakes and assembled films. Progress in Materials Science, 120, p.100757. doi.org/10.1016/j.pmatsci.2020.100757
Disclaimer: The views expressed here are those of the author expressed in their private capacity and do not necessarily represent the views of AZoM.com Limited T/A AZoNetwork the owner and operator of this website. This disclaimer forms part of the Terms and conditions of use of this website.