Sponsored by MerckJan 22 2024Reviewed by Emily Magee
Lateral flow assays, also called immunochromatographic assays, identify or measure biomolecules in intricate samples like blood, saliva, urine, or other fluids to diagnose numerous medical conditions.1
They are commonly self-contained, portable tools known for their user-friendliness, speed, and affordability.
Lateral flow test devices can be kept at room temperature, boasting a long shelf life, and delivering diagnostic results without the need for complex sample handling or additional equipment. This makes them perfect for both point-of-care and field-based diagnoses.
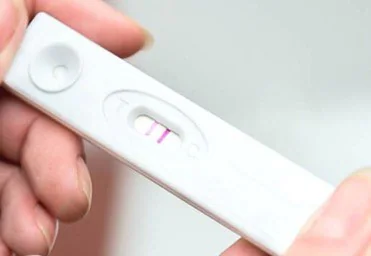
Figure 1. Image of commonly used lateral flow rapid test for determining pregnancy. Image Credit: Merck
Each year, countless lateral flow tests are used across various applications, including the well-known pregnancy test (Figure 1).
The sensitivity of these tests relies on the components utilized, the treatment of the components and samples, and the features of the tiny reporter particles responsible for generating the diagnostic signal.
The reporter particles are labeled with a molecule, frequently a nucleic acid or antibody, which identifies the analyte in the sample and attaches to a specific spot on the strip.
To enhance the assay's sensitivity, reporters are selected to be as "bright" as possible, producing a substantial signal intensity per binding occurrence. These reporter particles must be exceedingly small, usually on the nanoscale, to effectively bind with molecular targets.
Lateral flow assays comprise a sequence of cheap, paper-like components arranged on a backing card for easy handling (Figure 2). These components typically include:
- Sample pad: Absorbs the sample, controls its distribution, and manages its flow onto the conjugate pad.
- Conjugate pad: Dispenses and dries nanoparticle-antibody conjugates, ensuring regulated release onto the nitrocellulose membrane following hydration.
- Nitrocellulose membrane: Immobilizes Control line and Test line reagents.
- Wicking pad: Ensures consistent capillary flow through the membrane, absorbs the applied sample, and prevents any backward flow.
Each component overlaps the previous one by a minimum of 1 mm, enabling the sample to flow smoothly from the sample pad to the wicking pad without obstruction.

Figure 2. Schematic of a lateral flow test strip. Image Credit: Merck
In a lateral flow rapid test, a liquid sample like blood, plasma, serum, saliva, urine, or solubilized solids is applied to the sample pad. Capillary action pulls the sample through the lateral flow device.
Sometimes, the sample pad is treated with reagents to alter the sample's pH. It may alternatively contain filters to eliminate unwanted particles, such as red blood cells.
The sample moves from the sample pad to the conjugate pad, which holds dried, vividly colored, or fluorescent nanoparticles with antibodies attached to their surface. As the sample reaches the conjugate pad, the nanoparticles get rehydrated and mixed with the sample.
This mixture proceeds through the nitrocellulose membrane, passing across one or more test lines and a control line. These lines consist of fixed proteins that interact with the sample, producing a signal. The signal's strength corresponds to the quantity of analyte or conjugate present.
The remaining liquid gets absorbed by a wicking pad designed to trap the sample and prevent reverse flow. Once the sample has completely passed through, the signal contrast at the test line(s) can be interpreted visually or by using a reader to detect the presence of the analyte, and thereby the results.
Lateral Flow Assay Formats
The two primary formats for lateral flow assays are "sandwich" and "competition" (Figure 3).
The sandwich format is typically employed for identifying larger analytes that possess at least two binding sites, known as epitopes. Usually, one antibody to an epitope is linked to the nanoparticle (serving as the detection antibody on the reporter particle), while another antibody to a different epitope is utilized for the test line in the assay (capture antibody).
If the sample contains the analyte, it binds to both the detection and capture antibodies, forming a sandwich between the nanoparticle conjugate and the test line, resulting in a positive signal.
If the analyte is absent, the nanoparticle conjugate does not bind to the capture antibody, resulting in no observable signal. In sandwich assays, the intensity of the signal on the test line directly correlates with the amount of analyte present in the sample.
The competition format, on the other hand, is employed to detect analytes in cases where antibody pairs are unavailable or when the analyte is too small for multiple antibody binding events, such as small organic molecule drugs or steroids.
In this approach, the test line typically holds the analyte molecule, ordinarily a protein-analyte complex. If the target analyte is in the sample, it attaches to the reporter particle, preventing its binding to the analyte embedded within the test line.
When no analyte is present, the reporter particles attach to the analyte embedded in the test line, generating a signal. With competitive assays, the signal strength decreases with an increase in the amount of analyte present in the sample.
In both assay setups, an anti-species antibody serves as the control line, binding the conjugated antibody regardless of the test line outcome. The presence of a control line confirms the proper flow and functionality of the assay.
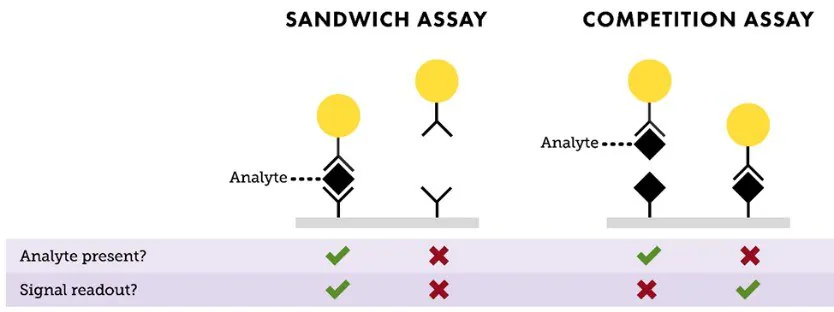
Figure 3. Depiction of “Sandwich” and “Competition” assay formats. In a sandwich assay, a positive signal indicates the presence of analyte, and the test line intensity is proportional to the amount of analyte available in the sample. For a competition assay, a strong signal on the test line means little or no analyte is present. This signal intensity is inversely proportional to the analyte concentration. Image Credit: Merck
Nanoparticles as Reporters in Lateral Flow
Lateral flow tests create an optical signal using vividly colored or fluorescent particles that are fixed to test lines on a white nitrocellulose strip. This signal can be interpreted visually (qualitative or semi-quantitative) or through an optical reader (quantitative).
To enhance test sensitivity, each binding event between an analyte and reporter particle should generate the most robust signal achievable.
While larger particles usually offer a stronger signal per binding event, excessively large particles struggle to flow smoothly through the nitrocellulose membrane and have limited chances to bind to the test line.
Hence, particles ranging between 20 nm and 500 nm in diameter are typically chosen for lateral flow assays. Fluorescent particles can also be used, following the same principles: higher fluorescence per binding event results in a more potent signal.
Gold nanoparticles are frequently employed as reporter particles in diagnostic assays and are often called gold colloids.2 Gold nanoparticles possess unique optical characteristics that make them excellent light absorbers.
For instance, gold nanospheres with a diameter of 40 nm strongly absorb light at 520 nm wavelengths, resulting in a test line appearing ruby red. The gold surface naturally attracts antibodies and other proteins, simplifying the creation of nanoparticle-antibody conjugates by merely combining gold nanoparticles with an affinity ligand.
Various sizes and shapes of gold nanoparticles have also been utilized as lateral flow probes. Gold nanoshells with a diameter of 150 nm offer greater contrast per binding event, typically resulting in a 5–20 fold increase in sensitivity compared to 40 nm gold nanospheres.
Gold nanoshells are prepped by the electroless deposition of gold onto the surface of a silica particle adorned with small gold nanoparticles.3 These small gold seeds gradually grow larger until they merge into a complete shell (Figure 4).
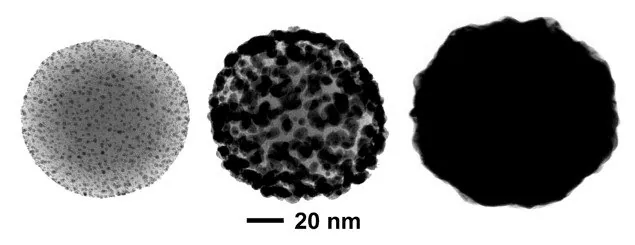
Figure 4. Transmission electron microscopy images of different stages of nanoshell growth. Initially, thousands of ~2 nm gold nanoparticle seeds are bound to positively charged silica nanospheres (left). Gold ions in solution are electrolessly deposited onto the bound gold seed, growing the seeds larger (middle) until they coalesce into a complete shell (right). Image Credit: Merck
By adjusting the size of the silica core and the thickness of the gold shell during the synthesis of gold nanoshells, it is possible to modify the particle's color based on its peak wavelength.
To enhance the sensitivity of lateral flow assays, blue-gray 150 nm gold nanoshells with a 120 nm silica core and approximately a 15 nm gold shell have been established. The silica core, being significantly lighter than gold, improves settling time and flow characteristics within the nitrocellulose membrane compared to solid gold particles.
Figure 5 illustrates a comparison between the optical extinction (scattering plus absorption) per particle of 40 nm gold nanospheres and 150 nm nanoshells. Due to their heightened extinction per particle, each nanoshell binding event presents a substantially higher contrast against the nitrocellulose substrate, thereby elevating the assay's sensitivity.

Figure 5. Per particle optical extinction (optical density per particle) as a function of wavelength for 40 nm gold nanospheres and 150 gold nanoshells. Image Credit: Merck
Figure 6 shows test and control lines utilizing gold nanoshell reporters, accompanied by a scanning electron microscope image. In the image, individual nanoshells bound to antibodies and then fixed onto the nitrocellulose membrane appear as light-colored spheres against the darker nitrocellulose fibers.
Despite the relatively low coverage density on the membrane, the control line appears markedly dark. This showcases that each nanoshell binding event generates a strong contrast signal against the white nitrocellulose background.
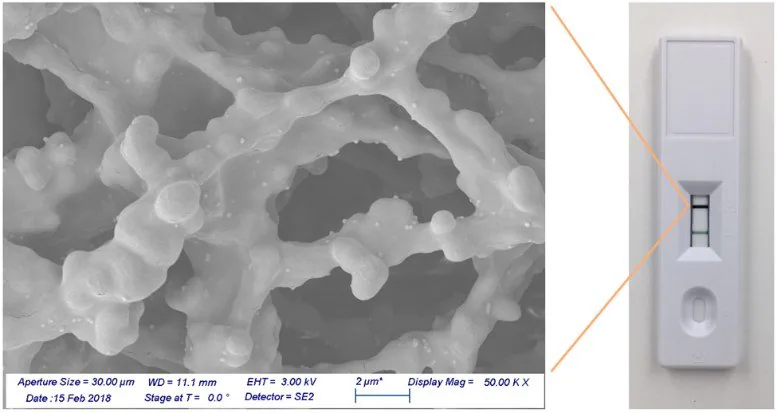
Figure 6. Gold nanoshells bound to a nitrocellulose membrane. Image Credit: Merck
The assay's sensitivity depends on the number of reporters needed to create a visible test line. Top of Form
To measure this, streptavidin-coated 40 nm gold nanospheres and streptavidin-coated 150 nm gold nanoshells were captured on a biotinylated test line, and the signal was assessed using an optical reader (as shown in Figure 7).
Using 40 nm gold nanospheres, a visible test line emerged when 5 million particles were added to the assay. However, with gold nanoshells, only 500000 particles were needed to visualize a line—a tenfold reduction, leading to heightened assay sensitivity.
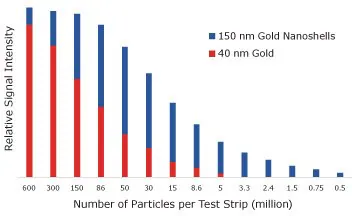
Figure 7. Lateral flow assay results where streptavidin-functionalized 150 nm gold nanoshells and 40 nm gold nanoparticles were placed on a nitrocellulose strip and captured on a biotinylated bovine serum albumin test line. The visual detection limit of gold nanospheres is 5,000,000, but for nanoshells, it is 500000 binding events. Image Credit: Merck
To assess how the absorbance per particle influences assay sensitivity, both 40 nm diameter gold nanoparticles and 150 nm diameter gold nanoshells were employed to detect Troponin I, a cardiac marker, in a lateral flow sandwich assay (Figure 8).
With 40 nm gold nanoparticles, the limit of detection was 0.5 ng/mL for Troponin I. With 150 nm gold nanoshells, the detection limit significantly improved by an order of magnitude to 0.05 ng/mL for Troponin I.
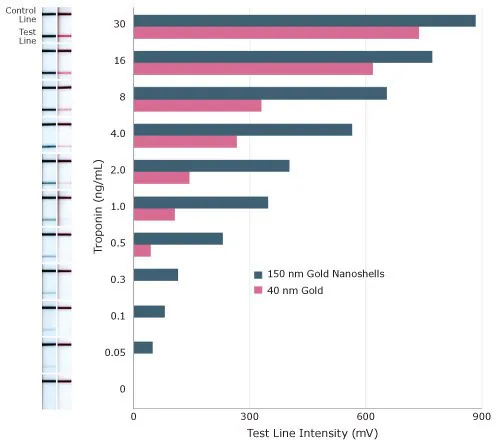
Figure 8. Sensitivity of lateral flow assay for Troponin I using 150 nm gold nanoshells and 40 nm gold nanospheres as reporter particles. Image Credit: Merck
Covalent Linkage Chemistry for High Stability Reporters
Another key factor to consider when developing high-sensitivity lateral flow assays is how antibodies or other proteins bind to the surface of nanoparticles. Establishing a robust and efficient binding process is essential for increasing the sensitivity and selectivity of the assay.
The process of attaching antibodies to the surface of a reporter particle is called conjugation, resulting in the formation of an antibody-coated particle known as a conjugate.4,5
Passive adsorption (physisorption) is the conventional method for linking proteins to lateral flow nanoparticle probes and remains widely used.
By exploiting the interactions between molecules and surfaces at a specific pH (such as van der Waals and ionic forces), antibodies can be forced to spontaneously bind to a bare gold nanoparticle surface to create a conjugate.
An excess of antibodies is typically added to ensure complete coverage of the nanoparticle surface. Any surplus antibodies remaining in solution post-conjugation are eliminated through centrifugation or filtration.
Alternatively, conjugates can be created by covalently binding the antibody to the nanoparticle surface. Covalent binding tends to offer more consistent results than physisorption. Moreover, for certain antibodies, covalent chemistry is necessary to produce stable conjugates.
Covalent binding chemistry offers several advantages, including increased control over the quantity of antibodies on nanoparticle surfaces and the orientation of the antibody.
Additional benefits of covalent binding are:
- Fewer antibody is required to enhance sensitivity, lowering overall costs.
- Greater stability of covalent conjugates, enabling their application in challenging sample matrices and high salt/detergent environments.
- Ease of preparation without extensive salt or pH adjustments, saving time in antibody screening experiments.
- Precise control over the antibody-to-particle ratio, which is crucial for adjusting the dynamic range in competitive assays and optimizing sensitivity with antibodies of varying binding kinetics.
One prevalent method for producing covalent conjugates involves utilizing carboxyl (carboxylic acid) functionalized gold surfaces.
A carboxylic acid on the nanoparticle can be linked to a primary amine found in the lysine residues of the antibody or protein using reagents like 1-Ethyl-3-(3-dimethylaminopropyl)carbodiimide (EDC) and N-hydroxysulfosuccinimide (sulfo-NHS) to form amide bonds.
An average IgG antibody typically contains 80–100 lysine residues, of which 30–40 are accessible for EDC/NHS binding.
Interpreting Results From Lateral Flow Tests
The outcomes of a lateral flow test can be qualitative (if an analyte is or is not present within the limits of detection), semi-quantitative (analyte present at low, medium, or high levels), to quantitative (precise amount of analyte present determined) assessments.
Qualitative tests determine whether an analyte is present within the limits of detection or not. An example is the pregnancy test, providing a simple "yes" or "no" result based on the presence or absence of elevated hCG hormone levels in urine—a positive test line correlates with the presence of hCG, indicating pregnancy.
In contrast, quantitative diagnostics involve comparing test line intensities to calibration standards, enabling the conversion of these intensities into specific analyte concentration values. Quantitative assays measure the precise concentration of an analyte or biomarker rather than solely indicating its presence or absence.
For instance, someone managing high stress might aim to accurately measure their cortisol levels over time to gauge the effectiveness of stress-reduction interventions.
To measure the test line intensity accurately, the result needs to be analyzed using a strip reader. This method enables accurate quantification of the analyte concentration in quantitative lateral flow assays.
The emergence of compact, affordable, and mobile-centric readers in the market is revolutionizing the lateral flow assay industry.
These recent advancements offer simple ways to quantify lateral flow assay outputs without requiring a standalone, bulky benchtop reader. This shift presents significant opportunities for home use and point-of-care diagnostics.
Figure 9 displays various disposable and benchtop reader formats that are currently available. Benchtop units typically involve inserting a lateral flow cartridge into the instrument, where a camera captures the intensities of the test and control lines. The captured results are then transmitted to a computer or mobile phone for display and interpretation.
The software within these systems can incorporate calibration curves specific to each assay. This allows automatic conversion of test line signal intensity to analyte concentration, providing users with precise information.
Disposable readers contain all the necessary hardware to conduct the analysis directly on the device itself, further simplifying the user experience.
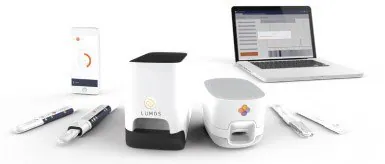
Figure 9. Various Lumos Diagnostic reader formats for lateral flow diagnostics. Image Credit: Merck
Conclusions
Ultra-bright reporter particles based on the distinct optical properties of gold nanoshells substantially elevate the sensitivity of lateral flow immunoassays.
These advancements, coupled with recent strides in portable reader technology, are introducing a novel category of point-of-care diagnostics to the market. These diagnostics exhibit sensitivity, specificity, and reproducibility akin to laboratory-based tests but within a much more cost-effective and convenient test format.
The application range of gold nanoshells extends beyond lateral flow assays to encompass diverse diagnostic and detection domains. The versatility proves valuable in surface-enhanced Raman spectroscopy, flow cytometry, molecular imaging, and other related fields.
The compatibility with simple covalent coupling chemistry and user-friendly protocols positions gold nanoparticle conjugates as essential tools across numerous biotechnology applications.
References and Further Reading
- Wong R, TSE H. 2009. Lateral Flow Immunoassay. New York, NY: Humana Press.
- Wilson R. 2008. The use of gold nanoparticles in diagnostics and detection. Chem. Soc. Rev.. 37(9):2028. https://doi.org/10.1039/b712179m
- Oldenburg S, Averitt R, Westcott S, Halas N. 1998. Nanoengineering of optical resonances. Chemical Physics Letters. 288(2-4):243-247. https://doi.org/10.1016/s0009-2614(98)00277-2
- Hermanson G. 2013. Bioconjugate Techniques. Academic Press.
- 2017. Nanomaterial Bioconjugation Techniques. MilliporeSigma.
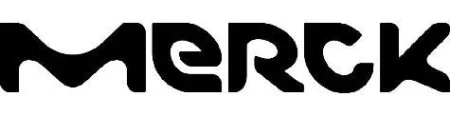
This information has been sourced, reviewed, and adapted from materials provided by Merck.
For more information on this source, please visit Merck.