Spectroscopic techniques involve the interaction of light with matter, which makes them powerful tools for investigating the properties of optoelectronic materials. This article explores the realm of spectroscopic techniques that are utilized for optoelectronic material analysis, focusing on their operational principles and diverse ranges of application.
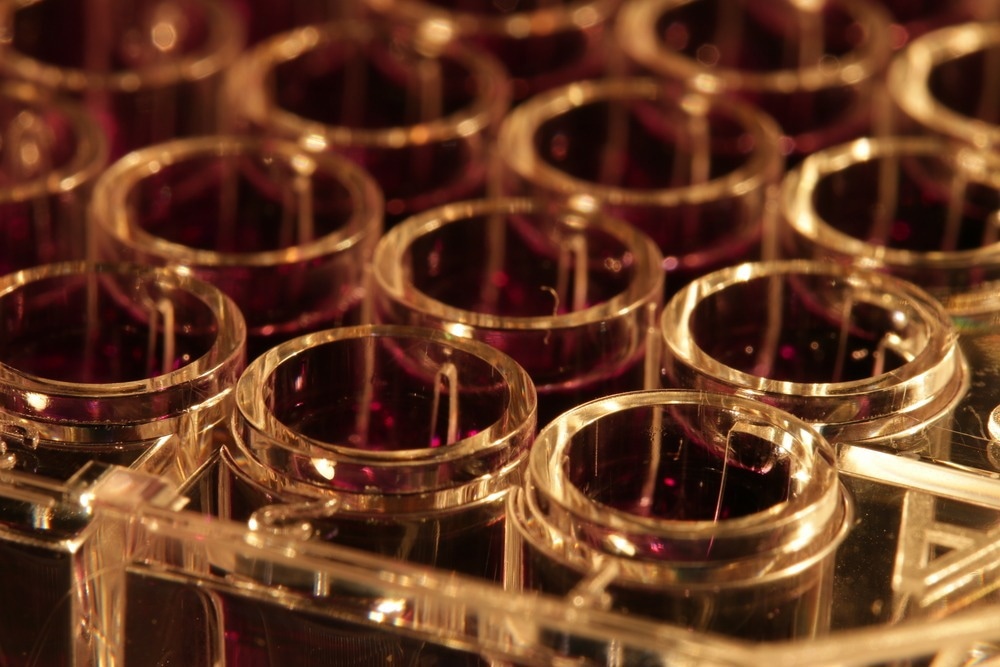
Image Credit: schlyx/Shutterstock.com
Optoelectronic materials, which exhibit properties of both electronics and optics, have revolutionized various technologies, including solar cells, light-emitting diodes (LEDs), and sensors. These materials have unique electronic and optical properties that make them essential for modern optoelectronic devices.
Depending on the material properties and light wavelength, light can be absorbed, transmitted, or reflected, allowing spectroscopic techniques to gather information about the electronic structure, chemical composition and physical properties. This analysis plays a vital role in understanding optoelectronics materials at the atomic, molecular, and electronic levels.
Various spectroscopic techniques are employed for optoelectronics materials analysis, including UV-Vis spectroscopy, photoluminescence spectroscopy, Raman spectroscopy, Time-resolved spectroscopy, Fourier-transform infrared spectroscopy (FTIR), and X-ray photoelectron spectroscopy (XPS), among others. In this article, we will primarily focus on the more common techniques and their practical applications.
UV-Vis Absorption Spectroscopy
UV-Vis spectroscopy is a widely used to analyze the electronic structure of materials. It involves the absorption of ultraviolet (UV) or visible (Vis) light exciting electrons to higher energy levels and providing insights into electronic transitioning occurring within the materials. UV-Vis spectroscopy is particularly useful for determining the bandgap of materials, which is a critical parameter for optoelectronic devices such as solar cells and LEDs. It can also provide insights into the presence of impurities or defects in the material, affecting its optoelectronic properties.
For instance, researchers at the University of Cambridge used absorption spectroscopy to study the electronic structure of hybrid perovskites, a class of materials that show promise for use in solar cells. They found that the electronic structure of these materials is highly sensitive to their chemical composition and the conditions under which it is synthesized.1
Photoluminescence Spectroscopy
Photoluminescence spectroscopy is another technique that measures the emission of light from a material after it has been excited by other light sources. When a material absorbs radiation, the energy is transferred to the electrons, exciting them to higher energy levels. When the electrons return to their original energy levels, they emit radiation in the form of light. The emitted radiation is related to the material's electronic and optical properties, including the bandgap energy, the carrier lifetime, and defect density.
Photoluminescence spectroscopy is a powerful tool for the study of optoelectronic materials, including semiconductors, quantum dots, and more. The technique can be used to measure the bandgap energy, which is essential for the design of solar cells and other optoelectronic devices. It can also be used to study the carrier lifetime of a material, which is related to the material's efficiency in converting light into electricity.
For example, K Nehra et. al, reported that the Photophysical features of phenanthroline ligands were analyzed through a photoluminescence spectrometer, which indicates a strong impact of the substituents on the photoluminescent properties of the materials.2
Raman Spectroscopy
Raman spectroscopy is a non-destructive technique that can provide information about the molecular vibrations and crystal structure of materials. Under this, a monochromatic light is scattered by a sample, and the frequency shift of the scattered light is measured. This frequency shift provides information about the molecular vibrations in the material, which is useful for studying the chemical composition, crystal structure, and phase transitions of optoelectronic materials. However, Raman spectroscopy is particularly useful for the analysis of organic materials, such as polymers and organic thin films, as well as for the characterization of nanostructured materials, such as quantum dots and nanowires.3
For example, researchers at the Indian Institute of Technology Madras used Raman spectroscopy to study the vibrational properties of perovskite solar cells. They found that the Raman spectra of these materials are highly sensitive to the crystal structure and composition of the material, which can be used to optimize the performance of these solar cells.4
In conclusion, spectroscopic techniques are essential tools for the analysis of optoelectronic materials. By providing information about the electronic and optical properties, these techniques enable researchers to optimize the performance of optoelectronic devices and develop new materials for future applications. The above-discussed techniques are just a few examples of the many spectroscopic techniques available for optoelectronic materials analysis, and their selection depends on the specific properties of the materials being studied.
References and Further Reading
Calvo, M. E. (2017) Materials Chemistry Approaches to the Control of the Optical Features of Perovskite Solar Cells. J. Mater. Chem. A, 5 (39), pp. 20561–20578. https://pubs.rsc.org/en/content/articlelanding/2017/TA/C7TA05666D.
Nehra, K.; Dalal, A.; Hooda, A.; Singh, D.; Malik, R. S.; Kumar, S. (2022) Spectroscopic and Optoelectronic Investigations of 3,8-Bis(3,4-(Ethylenedioxy)Thien-2-Yl)-1,10-Phenanthroline. J. Mater. Sci. Mater. Electron., 33(1), pp. 115–125. https://link.springer.com/article/10.1007/s10854-021-07268-5.
Madhuri, S. N.; Hemalatha, K. S.; Rukmani, K. (2019) Preparation and Investigation of Suitability of Gadolinium Oxide Nanoparticle Doped Polyvinyl Alcohol Films for Optoelectronic Applications. J. Mater. Sci. Mater. Electron., 30(9), pp. 9051–9063. https://link.springer.com/article/10.1007/s10854-019-01237-9.
Samji, S. K.; Tiwari, B.; Surendra, M. K.; Ramachandra Rao, M. S. (2014) Light Induced Phase Change in Cu2-XZn1.3SnS 4 Thin Films. Appl. Phys. Lett., 104(15), pp. 3–8. https://pubs.aip.org/aip/apl/article-abstract/104/15/152106/131073/.
Disclaimer: The views expressed here are those of the author expressed in their private capacity and do not necessarily represent the views of AZoM.com Limited T/A AZoNetwork the owner and operator of this website. This disclaimer forms part of the Terms and conditions of use of this website.