The convergence of nanotechnology and quantum science is rapidly expanding and proving to be mutually beneficial to both fields. It has sparked some of the most exciting scientific breakthroughs of the last decade, with even more on the horizon.
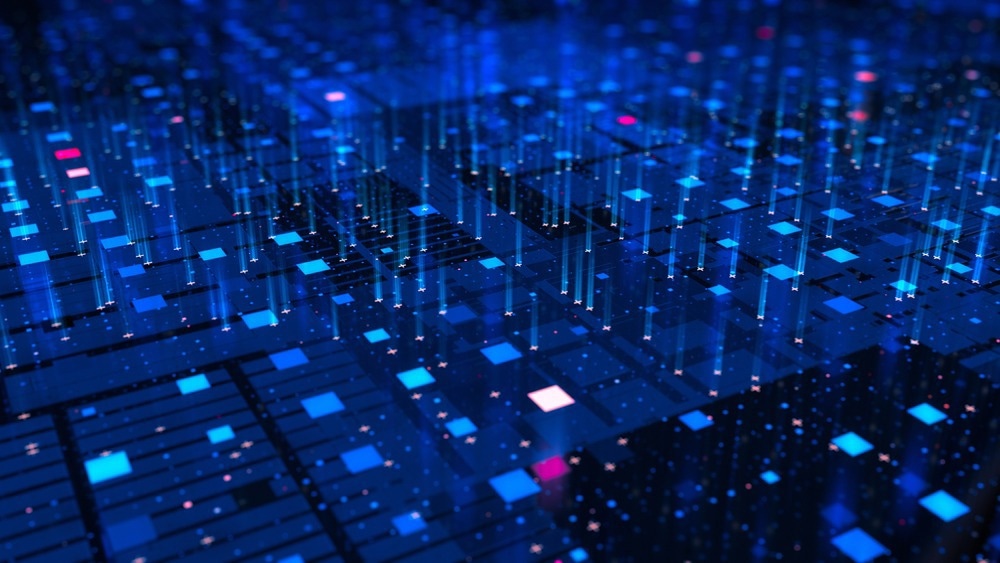
Image Credit: Untitled Title/Shutterstock.com
This article delves into the latest developments in crucial areas of quantum research, leveraging the enabling capabilities of nanotechnology while providing insight into what the future holds.
Nanotechnology and Quantum Physics
Quantum nanoscience is a vital research area that intersects nanoscale science and quantum science, providing essential insights for developing nanotechnologies. It leverages quantum mechanics to explore and exploit coherent quantum effects in engineered nanostructures, offering possibilities for new nanodevices and nanoscale materials.
The functionality and structure of quantum nanodevices are described through quantum phenomena like coherence, superposition and entanglement. This field has taken on new significance as quantum computing becomes a reality, unlocking the potential for revolutionary developments at the nanoscale.
Quantum phenomena are typically observable at smaller lengths and time scales than what we experience in our daily lives, typically involving individual particles or excitations. Advances in structuring matter at the nanoscale have made experiments at the single particle level commonplace, opening up opportunities to explore and harness quantum mechanical effects in condensed matter. These phenomena offer possibilities for new technological developments in communication, computing, and sensing the nanoscale world.
Nanotechnology encompasses technological developments that employ a section or part operating on the nanoscale, between 0.1 and 100 nanometers (nm), exploiting the laws of quantum mechanics.
Nanoscale materials and devices are used for quantum metrology and sensing, building blocks for quantum computing, and sources and detectors for quantum communication. They enable investigations into quantum behavior and unconventional states in nano- and opto-mechanical systems, low-dimensional systems, molecular devices, nano-plasmonics, quantum electrodynamics, scanning tunneling microscopy, and more.
Applying Quantum Coherence
Quantum science and technology have emerged as a cross-disciplinary research pursuit, connecting these same areas and boasting immense commercial potential. However, while quantum physics governs the behavior of nanoscale objects, quantum coherence, which is essential for quantum information, communication, and sensing, has not played a prominent role in nanotechnology developments.
Coherence, the property that enables the prediction of a quantum system's evolution in time once it has been prepared in a superposition of different states, is crucial for specific tasks, such as performing a sequence of logic operations in a quantum computer. But quantum coherence is fragile and can easily be lost if the system becomes too large or is subjected to uncontrolled interactions with the environment.
Quantum coherence-enabled functionality holds the potential to drive disruptive technologies, such as quantum computing, quantum communication, quantum simulation, and quantum sensing. Since coherent quantum effects at the nanoscale are still relatively unexplored, the field of quantum nanoscience provides an avenue into this uncharted frontier of knowledge.
This research field's objective is to manipulate and leverage quantum-coherent functionality while comprehending the mechanisms of decoherence to preserve and maximize coherence.
Quantum Computing with Spin States
Gate-defined quantum dots (QDs) in gallium arsenide (GaAs) have played a critical role in pioneering spin qubit devices due to their favorable electronic properties and relative simplicity of fabrication.
While spin qubits were first demonstrated in GaAs in 2005, comparable devices in silicon (Si) remain challenging. The operation of gate-defined spin qubits relies on voltages, making the quantum processor susceptible to effective electrical noise.
Further improvement in coherence and control fidelity will benefit from reduced charge noise and improved dynamic nuclear polarization procedures. While Si is often viewed as advantageous due to its compatibility with complementary metal-oxide-semiconductor (CMOS) processing, the unusual layouts needed for Si qubits will incur significant development costs.
Nonetheless, GaAs-based devices remain a workhorse for proof-of-concept quantum information processing, and advances in optical coupling hold considerable potential for future breakthroughs.
Manipulating Single Electrons for Quantum Metrology
The ability to generate quantized electric currents through single-electron control has significant applications in metrology and sensing. Current research is focused on developing single-electron pumps (SEPs) for use in primary quantum standards.
The main challenge is establishing the universality of the current-to-frequency relation, which requires manipulation of tunneling rates over many orders of magnitude while accounting for disorder and potential fluctuators.
Validation of current quantization at accuracies better than 10^-8 will be necessary to demonstrate this universality. To achieve this, device technology must be developed to maximize charging energy and sharp transients in the time-dependent tunneling rates.
While GaAs are presently the most reliable technology for high-accuracy SEPs, Si-based SEPs show potential for higher frequency by utilizing strong SE confinement in trap states.
Creating Quantum Light Sources
Light has both classical and quantum mechanical properties, with quantum correlations and photon statistics emerging as defining features of non-classical (quantum) light. Photonic quantum technologies primarily focus on these non-classical states of light, including single photons, squeezed states, and multiphoton entangled states.
Quantum light sources have undergone significant development in recent decades, with applications expanding beyond quantum secure communication to more complex photonic quantum systems and networks. While early research focused on single-photon emitters, it has since been realized that faint laser pulses combined with decoy-state protocols can also increase security with existing technologies.
Contemporary research in quantum light sources aims to address even more complex quantum technologies, such as secure long-distance quantum communication with quantum repeaters, and photon-based quantum information processing like photonic quantum simulators and photonic quantum computers. Achieving these goals will require significant theoretical and experimental efforts, as no physical system currently fulfills all the requirements.
Despite significant scientific progress in understanding the properties of various physical systems, there remain serious challenges in translating this research into real-world applications. To achieve efficiency enhancement, scalability, miniaturization, and cost-reduction, it is necessary to apply industry-like engineering efforts to quantum light source technology.
References and Further Reading
Critchley, L. (2018). Quantum Technology and Nanotechnology. [Online] AZO Quantum. Available at: https://www.azoquantum.com/Article.aspx?ArticleID=82
Flamini, F., et al (2018). Photonic quantum information processing: a review. Reports on Progress in Physics. doi.org/10.1088/1361-6633/aad5b2.
Laucht, A., et al (2021). Roadmap on quantum nanotechnologies. Nanotechnology. doi.org/10.1088/1361-6528/abb333.
Heinrich, A., et al (2021). Quantum-coherent nanoscience. Nature Nanotechnology. doi.org/10.1038/s41565-021-00994-1.
Pekola, J.P., et al (2013). Single-electron current sources: Toward a refined definition of the ampere. Reviews of Modern Physics. doi.org/10.1103/RevModPhys.85.1421.
Quantum nanoscience. (2021) Nature Nanotechnology. doi.org/10.1038/s41565-021-01058-0.
Yamahata, G., et al (2017). High-accuracy current generation in the nanoampere regime from a silicon single-trap electron pump. Scientific Reports. doi.org/10.1038/srep45137.
Disclaimer: The views expressed here are those of the author expressed in their private capacity and do not necessarily represent the views of AZoM.com Limited T/A AZoNetwork the owner and operator of this website. This disclaimer forms part of the Terms and conditions of use of this website.