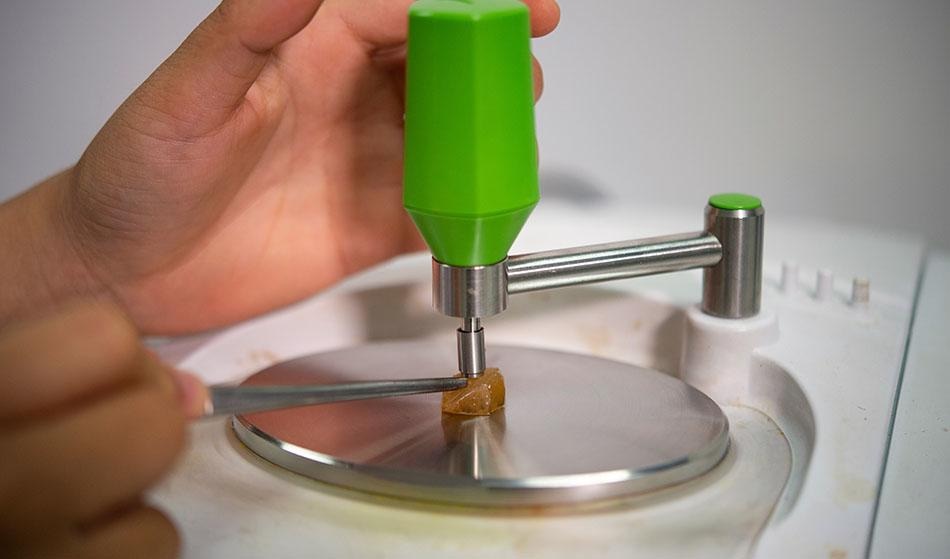
Rabbitmindphoto / Shutterstock
Infrared (IR) spectroscopy and Fourier transform IR (FTIR) spectroscopy are well-established techniques that are used across many industries. But like any characterization method, new advances continuously occur; often arising from the combination of different techniques. One method to emerge in this way is nanoscale FTIR, which takes principles from FTIR spectroscopy, atomic force microscopy (AFM) and scattering-type scanning near-field optical microscopy (s-SNOM).
The ability to analyze a material or substance with a nanoscale resolution is becoming even more important as we start to shift from using bulk materials to nano and micron-sized materials. But to use these materials, the topographical structure and properties need to be known to ensure correct characterization and efficiency. Here we’re going to look at how and why nano-FTIR is starting to be used for analyzing materials at the nanoscale.
Nano-FTIR Principles
The principles of AFM and s-SNOM feature heavily in the operating procedure of nano-FTIR. Nano-FTIR uses a metal-coated AFM tip to scan the surface of a sample, where a beam of IR light is shone from a light source through a beam splitter and a parabolic mirror, and on to the tip. The main difference between s-SNOM and nano-FTIR is the beam of light used, where nano-FTIR uses a much broader light beam. The shining of the light on to the tip causes the light to illuminate it due to the interactions between the metal coating and the light. This interaction also causes a strong near-field.
The tip scans and operates much like the conventional AFM and s-SNOM modes, in that the tip scans the surface and interacts with the sample—and these interactions give the location of each atom that the tip has interacted with, as well as some of its property or structural features. The difference with nano-FTIR over other methods is that the generated near-field interacts directly with the tip when it interacts with the sample, and this affects the backscattering process of the light during the analysis—as it is the backscattering of light to a detector which analyzes where the atoms are on the surface. The detector detects the backscattered light interferometrically and this yields a near-field interferogram of the surface.
However, because the sample is placed in one of the interferometer arms (rather than outside the interferometer as in FTIR), the near-field signal is boosted, removing the background noise while recording both the amplitude and phase of the light. This produces an FTIR spectrum that can be compared against known databases (but at a greater spectral resolution than conventional FTIR).
Benefits of Using Nano-FTIR
The ability to scan the surface with nanoscale resolution is of great benefit to anyone performing a surface analysis on a material or substance. The nanoscale resolution arises from the instrument performing hyperspectral imaging on the material by analyzing both the phase and amplitude of the detected light. This differs from FTIR which doesn’t analyze the phase information of the detected light. This enables a spectrum to be produced for every pixel of the image/scanned area, and because an atomically sharp tip is used to analyze the surface, each of these pixels has a nanoscale resolution that can provide a lot of information about the surface of the material and its properties. The average spatial resolution that is achieved using nano-FTIR is between 10 and 20 nm.
One other distinct feature of nano-FTIR over FTIR and similar methods is that it uses a broadband IR light source, and this enables it to produce IR spectroscopy analysis on very small quantities of materials, including on single molecules and monolayer nanomaterials.
Nano-FTIR can also be used on a wide range of materials. For many organic molecules, polymers and biological materials, the spectra can be simply compared to a known database, which leads to quick and accurate identification of these materials. However, the analysis of some of these softer materials is only possible because no sample preparation is required and the technique itself is non-destructive—unlike other techniques where the operating principle or sample preparation methods can destroy softer samples. It is a technique that is often used in conjunction with other methods to provide complementary data, but unlike the name suggests, it can provide information from the terahertz (THz) to the visible spectral range, making it a very versatile analysis technique—hence its greater use in recent years on the nanomaterials and thin materials that are becoming ever more popular.
Sources
Disclaimer: The views expressed here are those of the author expressed in their private capacity and do not necessarily represent the views of AZoM.com Limited T/A AZoNetwork the owner and operator of this website. This disclaimer forms part of the Terms and conditions of use of this website.