In this interview, AZoNano talks to Dr. Madeline Dukes, Application Scientist from Protochips about what is in situ TEM and why it is important for researchers.
What is TEM and why do people use it?
Transmission Electron Microscopy, or TEM, is a microscopy technique that uses a beam of electrons instead of light to form the image. Electron microscopy enables specimens to be imaged at much higher resolutions than light microscopy, due to their smaller wavelength. Thus, we can use it to image nanoscale specimens even down to the atomic level.
In order to maintain a coherent, high quality beam of electrons, the microscope column must maintain a high vacuum environment. This traditionally limits the types of samples that can be imaged, as the sample must also be stable under high vacuum. Electrochemists often use conventional TEM techniques to study the structure of electrode materials, pre- and post-experiment.
However, conventional TEM imaging does not provide kinetic or dynamic information. Technological advances over the last decade have led to the development of in situ TEM techniques.
What is in situ TEM, and why is this so important?
In situ techniques enable researchers to interact with the specimen by applying stimuli to samples directly inside the electron microscope. This allows us to study dynamic, changing systems and use the electron microscope as a real-time nanoscale laboratory to fill in the missing steps about dynamic, evolving processes that we can't observe by any other means.
An easy analogy to understand in situ TEM is an example from photography. Our eyes are not fast enough to discern the movement of a horse's hooves as it runs. Early, pre-photography paintings and drawings depict horses at full gallop with all four feet simultaneously off the ground.
It was not until 1878 that the missing pieces of the mechanism were captured. Photography showed for the first time that at no point during the horse’s movement, were all four feet off the ground. Likewise, in situ TEM allows us to fill in the missing gaps in evolving processes at resolutions we can't see using other methods.
Why has it been challenging in the past to use TEM for imaging wet and liquid samples?
TEM enables much higher resolution imaging than other techniques, such as light microscopy. Until the last decade the challenge in using TEM for wet samples is that a high vacuum is required for the electron microscope to operate. This means samples typically have to be dry in order to maintain the column vacuum and samples that are in liquid or hydrated states have to be processed either by drying, embedding in resin, or flash freezing before they can be imaged using TEM techniques.
So, how do we safely image wet samples in the TEM? The solution is to isolate the liquid between a pair of microchips which Protochips calls E-Chips. Each of these E-Chips contains ultra-thin, electron-transparent membrane that isolates the sample from the vacuum and forms an in situ liquid cell.
This enables us to safely image nanoscale samples in their native liquid environment, preserving not only their hydrogen structure but their dynamic behavior as well.
How has in situ TEM helped in the analysis of core-shell catalyst degradation?
In situ TEM was used to study the degradation mechanism of a core-shell platinum-palladium nanocatalyst particle (Shan 2018). These nanoparticles show very promising catalytic activity, but when integrated into fuel cells retain only 12 to 36 % of their activity.
Conventional TEM imaging of these particles pre- and post-experiment shows structural degradation and loss of surface area, which may be attributable to the loss of activity . Using in situ liquid cell TEM, researchers were able to observe and follow the degradation of individual catalyst particles in real time.
This allowed researchers to pinpoint how different types of defects in the particles’ platinum shell made them susceptible to different corrosion mechanisms. They identified three categories, color coded here: orange – regular cubs with no defects, blue – defects in the terrace of the cube and green – defects in the cube’s corners.
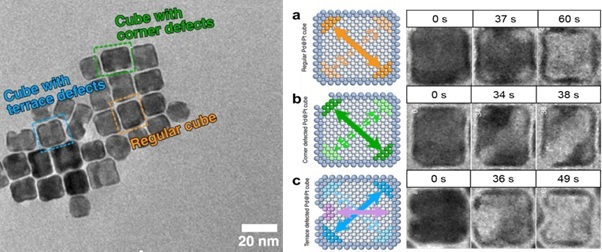
Image Credit: Protochips
The nanocubes that had a pristine platinum shell structure, with no defects, underwent a slow galvanic corrosion process, and the platinum shell remained structurally intact after etching away of the palladium core, and consequently, they retained their catalytic activity.
However, if the platinum shell had defects in either the corners or along the terrace, the internal palladium core is exposed to the electrolyte, and a much faster halogen etching processed occurs, resulting in the collapse of the cell shell structure due to the pre-existing defects and a significant loss of catalytic activity.
In this study, in situ liquid cell TEM provided a much more complete understanding of the factors that contributed to the overall degradation of catalytic activity when the nanoparticles were incorporated into a fuel cell.
How can in situ TEM benefit electrochemistry applications?
For electrochemistry applications, in situ TEM provides an unprecedented opportunity to supplement traditional ensemble measurements with time resolved imaging of evolving processes under operating or operando conditions.
By integrating electrochemistry into in situ liquid TEM experiments, we can explore a variety of different applications, such as the formation of the solid electrolyte interphase layer and dendrite growth in batteries, the ageing and degradation of nanoparticles for catalysis and environmental effects on corrosion processes.
What makes the Poseidon from Protochips such an ideal instrument for in situ TEM?
In order to transmit the electron beam through the sample, TEM specimens must be thin, ideally on the order of several hundred nanometers or less. This is true for in situ liquid imaging as well. Thus, it is necessary to scale down from traditional bulk volumes on the order of 10s of milliliters to sub-nanoliter in order to work within the transmissive and spatial confines of the electron microscope.
Specimens are inserted into the electron microscope using a dedicated in situ TEM holder, the Protochips’ Poseidon Select.. The liquid from the sample and the electrolyte must be hermetically sealed between the microchips called E-Chips, which support the electron-transparent membrane.
The Poseidon Select system lets you safely insert liquids and hydrated samples into the TEM to perform a wide variety of in situ experiments.
Within the sample holder, a single sealing gasket sits between the E-Chips and the body of the holder. A pair of chips enclose the sample and the seal is formed when the lid is attached, and the gasket is compressed. Integrated micro-fluidics and electrical contacts enable the delivery of liquids to the imaging region throughout the experiment, as well as stimuli for electrochemistry and temperature control.
Poseidon Select system is optimized for electrochemical performance inside the TEM. The unique miniaturized environment required for successful experiments means that Poseidon is optimized for low noise and ultra-low electrical measurements as evidenced in this platinum/sulfuric acid cyclic voltammetry plot (Holtz 2014) the electrochemical measurements are not appreciably affected by the TEM’s electron beam. The red line indicates that the beam is on and the black line is the same experiment with the beam off. Accurate, reliable delivery of fresh electrolyte to the active area is critical when working with such small volumes, to avoid electrolysis of the electrolyte over the course of the experiment.
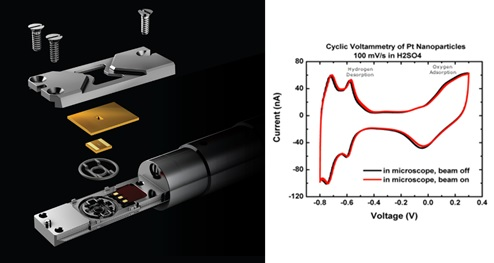
Image Credit: Protochips
The design of Protochips’ E-Chips isolates the electrochemically active area where the reaction is happening to the viewing window so that you don't miss any part of your experiment.
Fluid exchange between water and dilute sulfuric acid within the cell was tracked using Electrochemical Impedance Spectroscopy or EIS. Water was flowed into the cell, exchanged with sulfuric acid, and flushed again with water. This process was repeated five times, and the change in the impedance was measured using EIS to demonstrate the exchange of liquid.
How effective is the Poseidon Select for studying electrochemistry?
To give an example, a typical electrochemistry experiment - a ferricyanide/ferrocyanide redox couple - shows the sensitivity of the system (Unocic 2014).
Cyclic Voltammetry (CV) experiments provide insight into mass transport and diffusion-controlled processes.
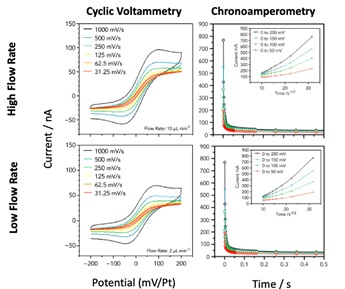
Image Credit: Protochips
These measurements, performed with the Protochips Poseidon Select, exhibited characteristic oxidation and reduction peaks when using fast scan rates, due to mass transport being dominated by diffusion, while at slower scan rates a sigmoidal profile occurred since diffusion is competitive with the timescale of the experiment. Using chronoamperometry, diffusion coefficients measured during the in situ experiments are consistent with the reported values as well as the CV curves.
Can the Poseidon Select be used to analyze batteries?
Yes. Batteries, particularly lithium ion batteries, power not only our cell phones and other consumer electronic devices, but more complex infrastructure as well, resulting in a $30 billion industry. However, when lithium batteries experience failure, this is not only costly but a significant safety hazard.
Research into developing safer, greener and more efficient lithium-based battery materials is a top priority among research labs throughout the world. One of the key factors that affects a lithium ion battery’s performance is the formation of its solid electrolyte interphase (SEI) layer, which forms along the interface of the electrolyte and the electrode.
If the SEI layer is unstable, it can develop cracks and healing features as it expands and contracts over multiple potential cycles.
These defects can result in the build-up of lithium dendrimers on the surface of the electrode over time, reducing the battery's efficiency and potentially causing short circuits to occur.
Protochips’ Poseidon system offers unique working electrode materials, one of which - glassy carbon - is transparent to the electron beam, enabling you to deposit your sample directly on it without losing a resolution or contrast due to the electrode material.
In one example, researchers at Oak Ridge National Lab (Sacci 2015) studied the growth of the SEI layer over a series of potential cycles. This image series shows the growth of the SEI layer that forms between the electrode (on the left side of each image) and the electrolyte (on the right side of each image).
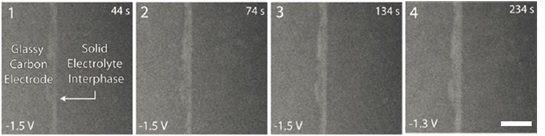
Image Credit: Protochips
The SEI layer is the light-colored line in the middle of each image, and its density and area increase with each subsequent potential cycle.
In a further example, researchers from the Nigel Browning and Layla Mehdi Group (Mehdi 2015) studied the charging and discharging process using lithium hexafluorophosphate. In this experiment, they are using a platinum electrode whose surface is clearly visible.
During the first charging cycle, as the potential is swept in the negative direction during the charging process, lithium electric deposition occurs, resulting in a build-up of lithium dendrimers on the surface of the electrode.
These dendrimers do not fully re-dissolve upon discharge as the potential is swept in the positive direction back into the electrolyte.
Cycle 2 shows the second charge/discharge cycle for the same cell at a lower magnification. During the second charging cycle, even greater amounts of lithium become trapped on the surface of the electrode due to the build-up of dendrites formed during the previous charge cycle.
This type of behavior is indicative of the structural changes that occur during capacity fading, since less lithium is re-dissolved into the electrolyte as the battery discharges, a shorter battery life results.
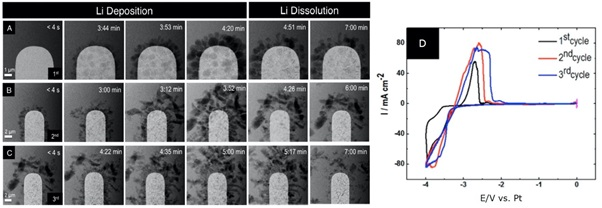
Image Credit: Protochips
Taking this a step further, in situ electrochemistry can be used to study the impact of small chemical changes on a battery's performance. In this next example, increasing the residual water content from 10 parts per million to 50 parts per million resulted in increasing concentration of lithium fluoride that forms during the electrochemical reaction (Mehdi 2016).
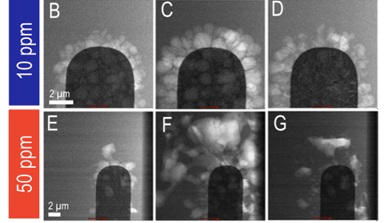
Image Credit: Protochips
This increase caused the formation of dendrimers with larger lithium grain sizes, but more efficient re-dissolution back into the electronic electrolyte during the discharge process, and a consequently better battery life.
How useful is in situ TEM within the fuel industry?
Fuel cells are another application ideally suited for characterization using operando TEM techniques. A $30 billion industry, fuel cells provide attractive environmental and transportation industry benefits. Development of catalyst materials that are durable and resistant to degradation is a key factor in developing robust commercial systems.
Electron microscopy is particularly well suited to the study of nanoscale behavior of catalyst materials during operating conditions. The development of new, more efficient catalyst materials is essential for wide adoption of fuel cell technology. Octahedral nickel-platinum catalysts supported on carbon electrodes exhibit high catalytic activity but degrade very quickly, limiting their commercial viability.
In this example, researchers studied the degradation of these octahedral catalyst particles under operating conditions to better understand the micro-structural changes to control their degradation.
Using the Protochips Poseidon Select, they applied both sequential potential cycles and periods of constant applied potential to replicate start-up and shutdown conditions.
A movie was obtained with in situ TEM, time-correlated with the applied electrochemical potential. Initially held at zero volts, the sample was then cycled 10 times. There was movement of the nanoparticles in the TEM image, but it was rather minimal at this point. The potential is then held again and followed by another 10 cycles.
The higher voltage cycles showed more movement of the particles. However, when we reached the final high voltage hold, we could see that significant mobility along the carbon support is occurring. During those potential cycles between zero and 1.2 volts, particle coalescence and particle motion were observed in the TEM images.
During the CV cycling, we could see some coalescence of the nanoparticles, as well as some movement of nanoparticles on the carbon support. However, when we moved into the anodic hold, we saw a complete rearrangement of the particles, and aggregation and agglomeration.
The results of this study found that the higher voltages used during the start-up and shutdown contributed more strongly to the degradation of the catalyst. Thus, developing nanocatalysts that are stable under these operating conditions is key to improving fuel cell performance.
In another example, researchers focused on a commercial fuel cell catalyst - Tanaka-TEC10V50E, a three nanometer platinum nanoparticle used in automotive applications.
Catalyst degradation is one of the primary limiting factors in the adoption of polymer electrolyte membrane fuel cells (PEMFC). By studying the cathode reaction in situ using Poseidon Select, scientists were able to monitor the evolution of the catalyst’s electrochemical active surface area to determine which structural degradation mechanisms were most impactful.
Cyclic voltammetry experiments were performed in situ up to 500 cycles to replicate normal stress testing protocols.
The platinum oxide reduction peak underwent a cathodic shift after the first 100 cycles, followed by an anodic shift. This indicated that the nanocatalysts underwent a reduction in size followed by subsequent growth.
Using energy filtering techniques, the researchers were able to push the TEM resolution in liquid below one nanometer, and they were able to link them to follow the different types of degradation processes hinted at in the electrochemical data.
These include detachment, coalescence, dissolution, growth and ostwald ripening. Corresponding with the sequential cathodic and anodic shifts observed in the platinum reduction peak, particle sizes measured from the TEM images followed the same pattern. Nanoparticles particles begin to dissolve and become smaller and then resume growth, following typical ostwald ripening mechanisms. In situ TEM enabled the researchers to understand, on a mechanistic level, the structural changes contributing to fuel cell ageing and degradation, that they also able to see in the electrochemistry ensemble measurements.
Are there any other areas where the Poseidon Select is especially useful?
Yes. Corrosion and corrosion management is a $2.6 billion industry. Corrosion affects our daily lives from maintaining infrastructure such as bridges and pipelines to our navies and shipping industries.
In situ liquid TEM enables us to look at this type of bulk scale process at the nanoscale level to further our understanding of early stage corrosion initiation, and development of corrosion resistant alloys and testing protocols.
When studying bulk materials, such as metals, using transmission electron microscopy, it is necessary to prepare a thin sample of the region of interest. This is traditionally achieved by preparing electro-polished thin film foils less than 100 nanometers thick. When performing in situ close cell studies, the spatial dimensions of these thin films must be further reduced.
This is done using a tool called a focused ion beam (FIB), which utilizes a stream of ions that essentially acts as a cutting torch. Thus, a small region of interest can be cut out of the foil and mounted across the membrane of the E-Chip for in situ liquid and electrochemical studies (Schilling 2017).
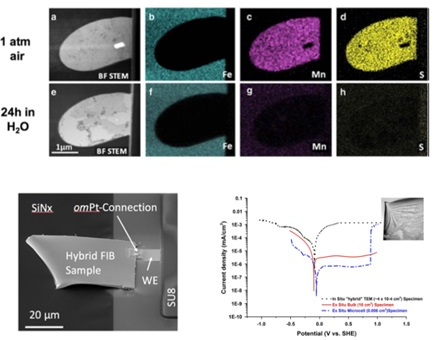
Image Credit: Protochips
As Poseidon Select is fully compatible with energy dispersive x-ray detectors, elemental analysis can be performed in liquid. In this example, energy dispersive x-ray spectroscopy was used to follow the dissolution of a manganese sulphide inclusion in stainless steel.
The inclusion is shown in the top row pre-experiment, where you can clearly see the manganese indicated in pink and the sulphur indicated in yellow.
The bottom row shows the same region after a 24 hour in situ experiment with exposure to water and the inclusion is fully dissolved with no manganese sulphide remaining.
An FIB section of type 304 austenitic stainless steel alloy is shown attached to the working electrode of the E-Chip, enabling measurements of potentiodynamic polarization curves in sulfuric acid for comparison to bulk data.
The black curve was measured in situ using Poseidon and the red curve was measured using traditional bulk methods. The peak for the corrosion potential correlates very well for both bulk and in situ data, with differences in current density attributed to the influence of charge build-up due to the electron beam.
How does the AXON software make in situ work more accessible?
One of the things that we have learned from working with our customers for over 10 years is that in situ electrochemistry experiments can seem intimidating, and that they may seem to require both expertise in electrochemistry and the knowledge of an experienced operator.
This year, we introduced AXON - a software package that redefines the in situ experience and makes in situ experiments possible for even a novice TEM operator.
AXON integrates the key equipment necessary for successful experiments and enables them to work seamlessly together using machine vision algorithms that intelligently identify, follow and correlate the features of the sample you've specified during the course of the experiment, making in situ experiments accessible like never before. AXON can be used with more than just Protochip systems and can enhance any dynamic or in situ TEM session.
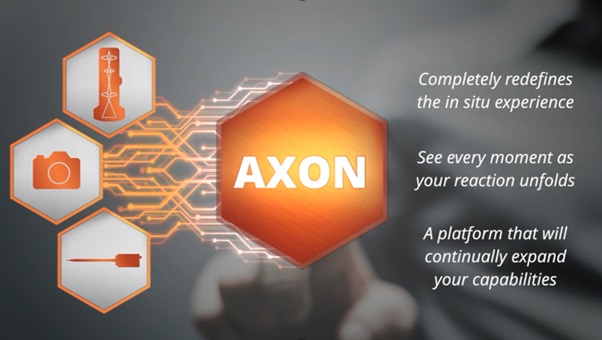
Image Credit: Protochips
For example, when performing a temperature ramp using a bulk furnace holder, heat expansion of both the samples and holder material cause significant thermal drift. With AXON off, applying a temperature ramp causes the field of view to drift signifcantly, requiring the TEM operator to make constant manual adjustments to bring the area of interest back into view, and eliminating the possibility of achieving atomic resolution.
With AXON on, the sample barely appears to move at all. AXON is doing the work for you, making constant on-the-fly adjustments of the stage, beam, and camera to keep the area of interest within the field of view at all times and allowing the operator to concentrate on the sample and obtain clear, high resolution images that were previously unobtainable during these type of experiments.
References
- Shan et al. Nat Communications, 9, 1011 (2018).
- Hotlz et al. Nano Lett. 14 (3), pp 1453–1459 (2014)
- Unocic et al. Microsc. Microanal. 20, 452–461 (2014)
- Sacci et al. Nano Lett.15 (3), 2011-2018 (2015)
- Mehdi et al. Nano Lett. 15 (3), pp 2168–2173 (2015)
- Mehdi et al. Scientific Reports, 6, 34267 (2016)
- Beerman et al. Energy Environ. Sci., 12, 2476-2485 (2019)
- ACS Appl. Energy Mater. 3, 3, 2360–2371 (2020)
- Schilling et al. Microsc. Microanal. 23, 741–750 (2017)
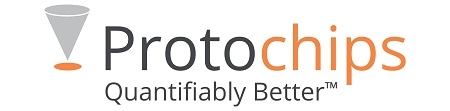
This information has been sourced, reviewed and adapted from materials provided by Protochips.
For more information on this source, please visit Protochips.
Disclaimer: The views expressed here are those of the interviewee and do not necessarily represent the views of AZoM.com Limited (T/A) AZoNetwork, the owner and operator of this website. This disclaimer forms part of the Terms and Conditions of use of this website.