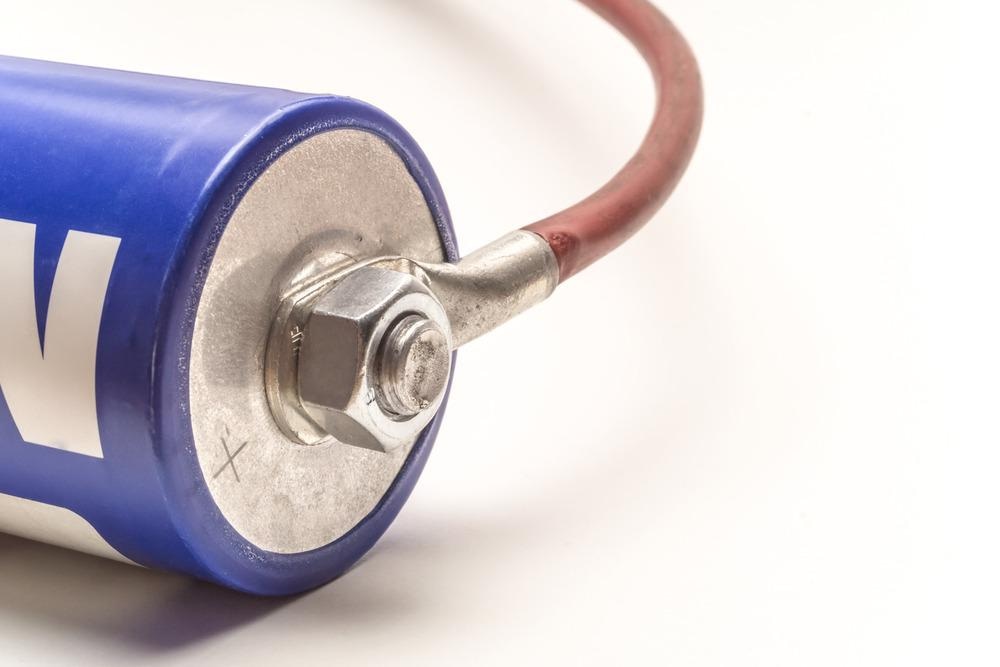
Image Credit: Dr. Me/Shutterstock.com
As a stand-alone material, graphene has some excellent electrical conductivity and charge carrier mobility properties that make it ideal for energy storage devices. However, for a lot of these devices, a single layer of graphene (often referred to as CVD graphene after the synthesis process) is required but compared to other types of graphene (multi-layer and graphene nanoplatelets), the cost of CVD is much higher, and it is not as scalable as other graphene production methods.
Other avenues are being utilized where graphene’s properties can be harnessed for energy storage devices. Hybrid materials offer different forms of graphene, including some exciting and novel architectures that conventional graphene manufacturing methods do not provide. One of the more novel approaches in recent years has been to combine graphene-based derivatives and turn them into metal-organic framework (MOF) structures.
The Development of MOFs for Energy Storage
MOFs themselves are hybrid materials, as they are made up of both organic and inorganic groups. MOFs are crystalline materials that have a regular and repeating network of pores throughout. These pores are possible thanks to a repeating unit of inorganic metal ions linked by flexible organic groups.
The metal ions and the organic groups both contribute to the size and shape of the pores in the MOF—something which is customizable to the intended application.
MOFs have found a lot of use in separation chemistry and are highly selective at storing different molecules. Outside of these areas, there is much potential for MOFs to be used as energy storage devices. However, MOFs are not the most conductive of materials, so making energy storage devices out of MOFs has had its inherent challenges.
Integrating Graphene Sheets into MOFs for Supercapacitors
Graphene has been touted as one of the materials that could help make MOF-based materials more conductive and suitable for supercapacitors. It is being used in many different ways in supercapacitor technologies.
When it comes to electrical conductivity, few materials outperform graphene, both in terms of the direct electrical conductivity and its charge carrier mobility—hence its introduction to energy storage devices.
While graphene is being used in supercapacitors, you have to be careful with its use. Capacitors work by having two conductive plates separated from each other. While its large surface area is another critical property that enables it to be used in supercapacitors, if the sheets get too close, then the van der Waals forces can cause the sheets to aggregate and form multi-layer graphene (and beyond). This is particularly relevant in supercapacitors, where the electric field produced by the supercapacitor gets stronger the closer the conductive plates are together (making it more effective at storing charge).
One way of preventing the graphene layers from coming together while harnessing their conductivity and large surface area has been to integrate functionalized graphene layers into MOF structures. Research to date has used many types of graphene sheets, from fluorinated graphene sheets to reduced graphene oxide.
Many of the hybrid graphene-MOF materials to date are not covalently linked and rely on a range of intermolecular interactions—such as π-π stacking and hydrogen bonds—to create a regular and stable porous network. While this provides the electrical conductivity properties required for supercapacitors, the materials' mechanical stability is not feasible for long-term use.
Creating Covalently Linked Graphene-MOF Supercapacitors
The latest research in this area has bucked the trend and opted for covalently linking the graphene sheets with the metal ions to create a much more mechanically robust hybrid supercapacitor. This was achieved by functionalizing the graphene sheets with specific organic groups to develop a ‘graphene acid’ sheet. The graphene sheets were then specifically attached to the inorganic MOF backbone (zirconium ions) to create a covalently linked graphene-MOF hybrid.
The chemical linking of the different constituents, rather than intermolecularly, not only provides more mechanical stability to material (and in turn the supercapacitor device), but it also enables the electronic properties of the material to be tuned.
The graphene acid sheet, in this instance, was linked with the zirconium ions via amine bonds, and the graphene-MOF hybrid displayed a large specific surface area, hierarchical pores, accessible interaction sites, and an interconnected conductive network. All the above properties are ideal for supercapacitor applications.
When functioning as a supercapacitor, the interconnected conductive network improved the rate capability and long cycling stability of the material. The amide linkages are thought to play a vital role in forming a π-conjugated structure, which helps to facilitate the charge transfer mechanisms and ultimately provide an excellent cycling ability and capacitance.
In terms of specifics, it was found that the material stored charge, with a capacitance up to 651 F g-1, which is much higher than many graphene supercapacitors. When the graphene-MOF was coupled to an opposing (negative) electrode made of a Mxene material, the device delivered a power density of up to 16 kW kg-1 and an energy density of up to 73Wh kg-1.
The device also showed capacitance retention of 88% after 10,000 cycles, showcasing its long-term stability and sustainable characteristics. Such approaches now offer a different route for creating effective, novel, and sustainable supercapacitors using advanced hybrid materials.
References and Further Reading
Science Daily [Online]. Available at: https://www.sciencedaily.com/releases/2021/01/210104131948.htm
Fischer R. A. et al. (2020) Covalent Graphene‐MOF Hybrids for High‐Performance Asymmetric Supercapacitors, Advanced Materials, https://doi.org/10.1002/adma.202004560
Disclaimer: The views expressed here are those of the author expressed in their private capacity and do not necessarily represent the views of AZoM.com Limited T/A AZoNetwork the owner and operator of this website. This disclaimer forms part of the Terms and conditions of use of this website.