The growing attempt to incorporate nanotechnology with electronics has resulted in the realization of emerging conceptual components and devices based on ferroelectric materials, such as transistors, memristors, capacitors, and many others.
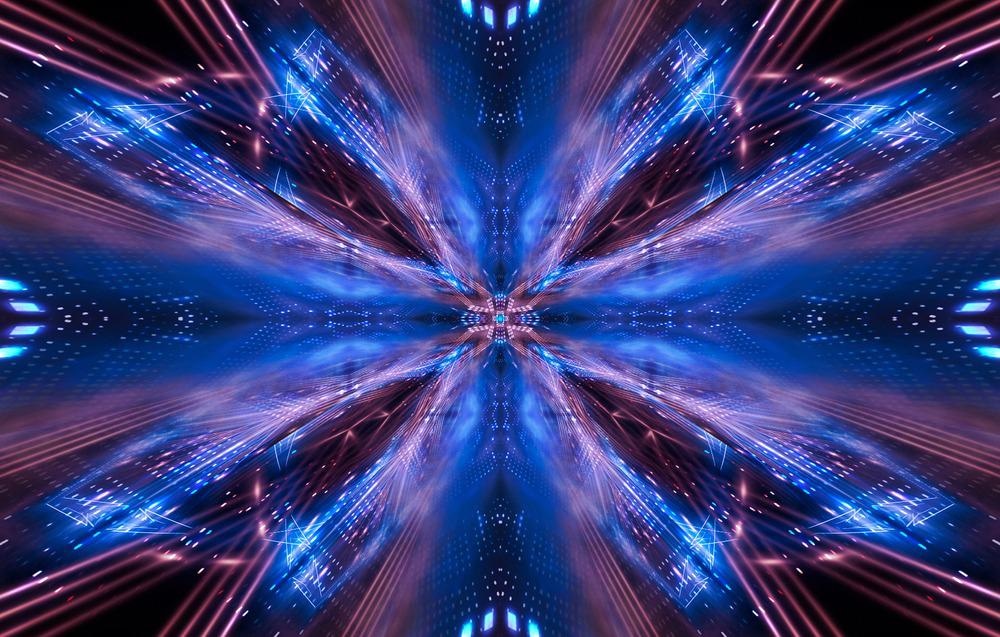
Image Credit: Mia Stendal/Shutterstock.com
In this article, an overview of ferroelectricity and its current development in the realm of ferroelectric-based nanoelectronics is given.
What is Ferroelectricity?
Opposite charges are attracted to one another and desire to combine in most materials, including metals. Their only movement is caused by the application of an electric field, which causes them to move to the opposite sides.
However, in ferroelectric materials, an external electric field is not required to maintain the separation of charges, resulting in spontaneous polarization.
The application of an external electric field, on the other hand, has an effect: an electric field in the opposite direction causes the charges to switch sides and reverse the polarization; an electric field in the same direction causes the charges to switch sides and remain polarized. This is why ferroelectric materials are used in a variety of electronic systems, such as those used in medical ultrasounds and radio frequency identification (RFID) cards, among other applications.
There are two variables that represent the fundamental aspect of any ferroelectric: the coercive electric field in correspondence to which the polarization vanishes and the remanent polarization, which represents the ferroelectric's intrinsic polarization when no electric field is applied.
The Properties and Benefits of Ferroelectric Materials
Even when the applied electric field is zero, ferroelectric materials exhibit spontaneous nonzero polarization. The nonlinear nature of ferroelectric materials can be used to create ferroelectric capacitors, which are used in ultrasound machines, infrared cameras, and other applications.
Ferroelectrics are distinguished by the fact that their spontaneous polarization can be reversed by applying a significantly strong electric field in the opposite direction, resulting in a hysteresis loop. This effect can be used as a ferroelectric RAM, computer memory function.
Ferroelectrics are all pyroelectric and piezoelectric, making ferroelectric capacitors extremely useful in sensor applications such as sonar, fire sensors, and vibration sensors.
Materials typically exhibit ferroelectricity only below a certain phase transition temperature, known as the Curie temperature (TC), and are paraelectric above this temperature.
Conventional Ferroelectric Materials
Ferroelectric materials can be structurally classified into three groups. The first is a compound containing hydrogen bonded radicals, in which proton motion is specifically associated with ferroelectric properties. Rochelle Salt and potassium dihydrogen phosphate are two examples (KH2PO4).
The second class of ferroelectric materials consists of ionic crystals with crystal structures similar to perovskite. BaTiO3, KTaO3, NaTaO3, KNbO3, PbTiO3(PZT), lead magnesium niobate (PMN), lead zirconate titanate (PZT), and others are examples.
The third category includes polymers and polymer composites such as PVDF.
Ferroelectricity and its Application in Nanoelectronics
Many applications in nanoelectronics are being driven by the recently discovered two main categories of ferroelectrics. The first is hafnium oxide (HfO2)-based ferroelectrics, which have been extensively researched as a gate dielectric in recent years due to their CMOS compatibility.
The traditional ferroelectrics (such as perovskites and PZT) are not CMOS compatible, and their ferroelectricity tends to disappear when they are thinned to the nanometer scale. HfO2-based ferroelectrics, on the other hand, are ferroelectric to a thickness of 1 nm.
2D ferroelectrics represent the second category. In this regard, graphene (a semimetal) and molybdenum disulfide (MoS2), a semiconductor, are two well-known examples.
How Ferroelectricity Could Change the Way We Store Data
Video Credit: Seeker/YouTube.com
HfO2-Based Ferroelectrics: Nanodevices Fabrication and Applications
To reduce the leakage current of billions of transistors integrated in a single chip, the relative dielectric constant must be increased (or relative permittivity). Doping HfO2 with elements such as yttrium and silicon promotes phase transition and the corresponding change in relative permittivity. For example, by doping HfO2 with zirconium oxide during atomic layer deposition, the tetragonal phase with the highest relative permittivity (app. 70) was obtained almost at room temperature.
Tunability of high-frequency components is critical for the advancement of future wireless communications, the Internet of Things and radar technologies. The high coercive fields of HfO2-based ferroelectrics enable massive tunability of microwave devices at only 1-3 V provided by a common battery.
For example, researchers from the National Institute for Research and Development in Microtechnologies Romania, created a microwave filter by growing a 6-nm-thick hafnium oxide doped with zirconium (HfZrO) layer on a 525-nm-thick Si substrate using atomic layer deposition (ALD) techniques. When the applied direct current (DC) voltage is swept between 0 V and 4 V, the filters are continuously tunable within the frequency range 0.1–16 GHz.
Another recently popular concept is the ferroelectric tunnel junction (FTJ), which consists of a nanometer-thick ferroelectric film sandwiched between metal electrodes. The thickness of the ferroelectric layer is thin enough to allow electron tunneling. Researchers designed and built a HfZrO-based tunneling diode with a top electrode made of 5 nm thick Chromium and 100 nm thick gold deposited on a 6-nm thick HfZrO thin film grown directly on doped silicon.
2D Ferroelectric Nanomaterials
Research into 2D ferroelectrics is on the rise thanks to the rapid development of nanotechnology. They could be used to make micro and nano-intelligent parts that have high performance and low energy consumption in the future.
Various 2D vdW materials, such as transition metal dichalcogenides (TMDs), MX2 (M = Mo W, X = Te, Se S), layer perovskites, metal triphosphates, indium selenide (In2Se3), and others, have been reported to be intrinsically ferroelectric; however, only a handful of 2D ferroelectric materials have been confirmed experimentally.
In 2018, researchers from King Abdullah University of Science and Technology reported that layered In2Se3 nanoflakes exhibit low-temperature ferroelectricity. Following that, a graphene/In2Se3 heterojunction was successfully used as a room-temperature ferroelectric diode, and in 2019, a MoTe2 2D nanostructure with ferroelectric properties was discovered at room temperature.
MIT researchers and colleagues recently discovered that the moiré superlattice created by sandwiching two graphene bilayers between atomically thin layers of boron nitride can be ferroelectric as well. One possible application is neuromorphic computation, which is based on circuits containing artificial neurons and synapses that mimic various brain functions like vision and learning.
Future Outlook on Ferroelectric Nanodevices
Ferroelectrics are finding increasing use in low-power nanoelectronics devices such as switches, negative capacitance transistors, and memristors. Although research in this area has just begun, started, real-world applications such as neuromorphic computation, fast processors, and reconfigurable systems for 5G and 6G communications are already significant.
References and Further Reading
Dragoman, M., Aldrigo, M., Dragoman, D., Iordanescu, S., Dinescu, A., & Modreanu, M. (2021). The Rise of Ferroelectricity at Nanoscale: Nanoelectronics is rediscovering the ferroelectricity. IEEE Nanotechnology Magazine, 15(5), 8-19. https://ieeexplore.ieee.org/document/9508206
Qi, L., Ruan, S., & Zeng, Y. J. (2021). Review on recent developments in 2D ferroelectrics: Theories and applications. Advanced Materials, 33(13), 2005098. https://onlinelibrary.wiley.com/doi/abs/10.1002/adma.202005098
Disclaimer: The views expressed here are those of the author expressed in their private capacity and do not necessarily represent the views of AZoM.com Limited T/A AZoNetwork the owner and operator of this website. This disclaimer forms part of the Terms and conditions of use of this website.