Ceramic nanoparticles are generally composed of metals and metal oxides, silicon carbide, nitrates, and carbonates like magnesium, chromium, and silicate. Because of their beneficial qualities, including strong heat tolerance and chemical stability, they have a wide variety of uses. There are a number of techniques widely used to synthesize nanoparticles of different ceramic materials.
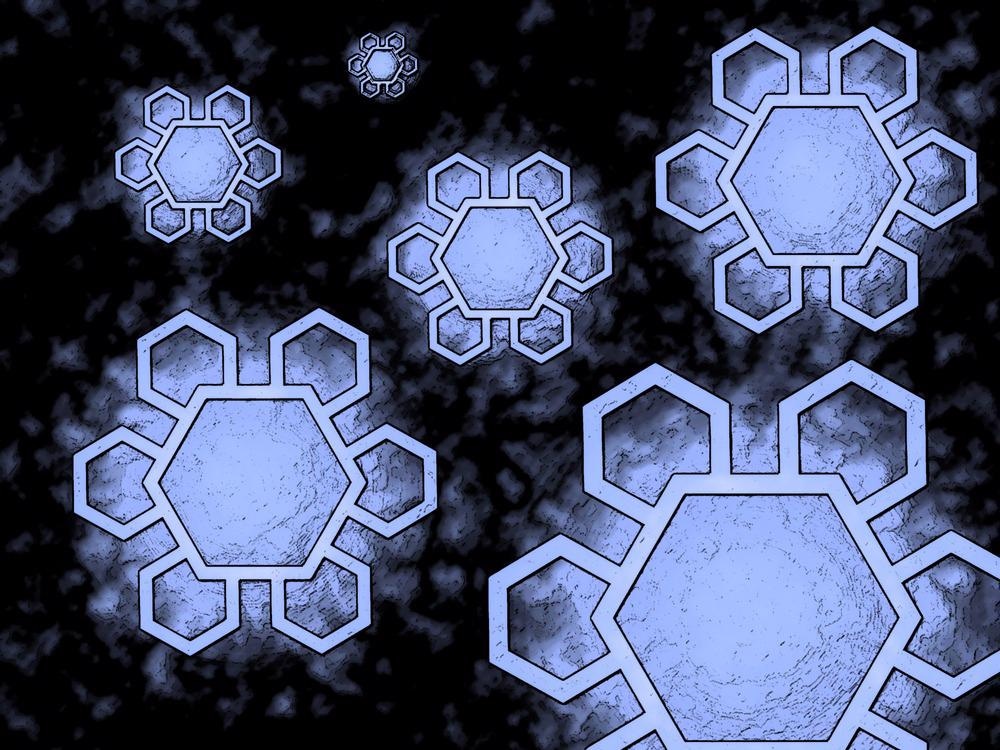
Image Credit: GiroScience/Shutterstock.com
An Introduction to Ceramic Nanoparticles
Ceramics are defined as having a definite solid core, arranged by the applying heat or even both heat and pressure, and consisting of a metallic and non-metallic mixture. Ceramic nanoparticles are made up of inorganic substances like aluminosilicate and are very prone to external disturbances.
Nevertheless, the nanoparticle center is not restricted to these two substances; instead, metals, metallic oxides, and metal sulfur compounds may be employed to create nanostructures of various sizes, forms, and permeability.
Advantages of Ceramic Nanoparticles
Ceramic nanoparticles have many benefits, including simple fabrication with appropriate size, form, and pores, and no influence on dilatation or permeability with pH values. The production of novel ceramic materials for biological applications has increased rapidly in recent years.
Controlled drug discharge is among the most explored areas of ceramic nanoparticles use in bioscience, where dosage and structure are critical. Long-term stability, relatively high loading capability, facile inclusion of hydrophilic and hydrophobic networks, and various delivery routes are all characteristics that make these nanostructures a promising tool in managing drug release.
Fabrication of Ceramic Nanoparticles
Usually, ceramic nanoparticles are created via solid-state processes. Raw ingredients such as oxide, hydroxide, nitrate, sulfate, or carbonate are physically combined and then processed at elevated temperatures for extended time spans to allow the nanoparticles to form. This process produces coarse-sized, aggregated nanoparticles with a reduced specific area.
The employment of elevated temperatures to create solid-state compounds often results in irregular grain development and a loss of stoichiometric control. Numerous modified chemical fabrication procedures have been devised to create ceramic nanoparticles with an acceptable shape at low temperatures.
Main Methods for Ceramic Nanoparticle Synthesis
The sol-gel procedure, also termed chemical solution deposition, is one method for creating nanoceramics. This comprises a liquid solution, or sol, composed of liquid-phase nanostructures and a predecessor, often a gel or polymer composed of particles submerged in a liquid.
The sol and gel are combined to form an oxide substance, which is a sort of ceramic, and the byproduct is vaporized. The majority of the nanoparticles are then warmed in a procedure termed densification to generate a quality product. This process might potentially be used to create a nanocomposite by burning the gel on top of a thin layer to build a nanoceramic coating.
Another way is two-photon lithography, which employs a laser to scrape a polymer into a three-dimensional pattern. The lasers strengthen the areas it hits but keep the remainder unhardened. The unhardened substance is then dispersed to form a "shell." The shell is then covered with ceramics, alloys, metallic crystals, and so forth. The final ceramic nanotruss may be crushed and returned to its original condition.
High-temperature sintering has also been utilized to solidify nanoceramic particles. This produced a rough substance that harmed the characteristics of ceramics and increased the duration required to produce a final product. This approach also restricts the final geometry that may be created. Microwave sintering was devised to address such issues.
A magnetron generates energy by vibrating and heating the particles with electromagnetic waves. Rather than transferring heat externally, this approach instantaneously transfers heat over the entire quantity of data.
Equipment for Ceramic Nanoparticle Synthesis
The apparatus for the fabrication is made up of many elements. For instance, the major component of the arrangement proposed by Rasche et al. (2020) is a three-zone heated flow tube reactor positioned vertically. It has a span of 6 m and can achieve temperatures of up to 1700 degrees Celsius. As individualized energy input into the separate zones is possible, the three-zone heating configuration promotes a homogenous temperature distribution.
The reaction zone for the synthesis method is an Al2O3 tube with an internal diameter of 105 millimeters. Because of its relatively strong heat transmission and damage tolerance, Al2O3 is an excellent option. Both sides of the tubes, and the fittings, are insulated with ceramics wool to prevent heat leaks and promote a consistent temperature distribution.
It must be noted that, in addition to reducing thermal losses, insulation contributes to a prolonged tube lifespan by preventing significant axial temperature differences. The processing gas is warmed to roughly 500 degrees Celsius for the same reason. To preserve the tube's longevity, heating and cooling rates must not exceed 300 K/h, which is accomplished by careful power regulation.
Problems with Synthesis of Ceramic Nanoparticles
Synthesis and processing are critical concerns in nanotechnology to harness the unique features of nanoparticles and realize their promising utility in research and technology. Many technical strategies for fabricating nanoparticles have been investigated.
There are some fundamental issues associated with the fabrication of ceramic nanoparticles utilizing any method or technology. These include a lack of controllability of nanoparticle size and structure, and the inability to manipulate the form of synthesized nanoparticles and particle size distribution. Moreover, the toxicity of the synthesized nanoparticles is also a key concern concerning real-world biomedical applications.
Addressing these concerns is critical in developing an optimal fabrication process for ceramic nanoparticles.
References and Further Reading
Rasche, D. B., Tigges, L., & Schmid, H.-J. (2020). An apparatus to synthesize ceramic nanoparticles with a precisely adjusted temperature history and a significant mass output. Review of Scientific Instruments. Available at: https://doi.org/10.1063/1.5133438
Singh, D., Singh, S., & Singh, M. R. (2016). Ceramic nanoparticles: Recompense, cellular uptake and toxicity concerns. Artificial Cells, Nanomedicine, and Biotechnology. Available at: https://doi.org/10.3109/21691401.2014.955106
Thomas, S. C., Harshita, Mishra, P. K., & Talegaonkar, S. (2015). Ceramic Nanoparticles: Fabrication Methods and Applications in Drug Delivery. Current Pharmaceutical Design. Available at: https://doi.org/10.2174/1381612821666151027153246
Disclaimer: The views expressed here are those of the author expressed in their private capacity and do not necessarily represent the views of AZoM.com Limited T/A AZoNetwork the owner and operator of this website. This disclaimer forms part of the Terms and conditions of use of this website.