Chemical reactions play a critical role in almost every aspect of everyday life. Most industrial catalytic processes require elevated operating temperature and pressure to ensure high yield. Recently, material scientists at Stanford University in the USA and the University of Cambridge in the UK developed a novel method based on nanophotonics that enabled them to exert much finer control over the catalytic activity of different materials.
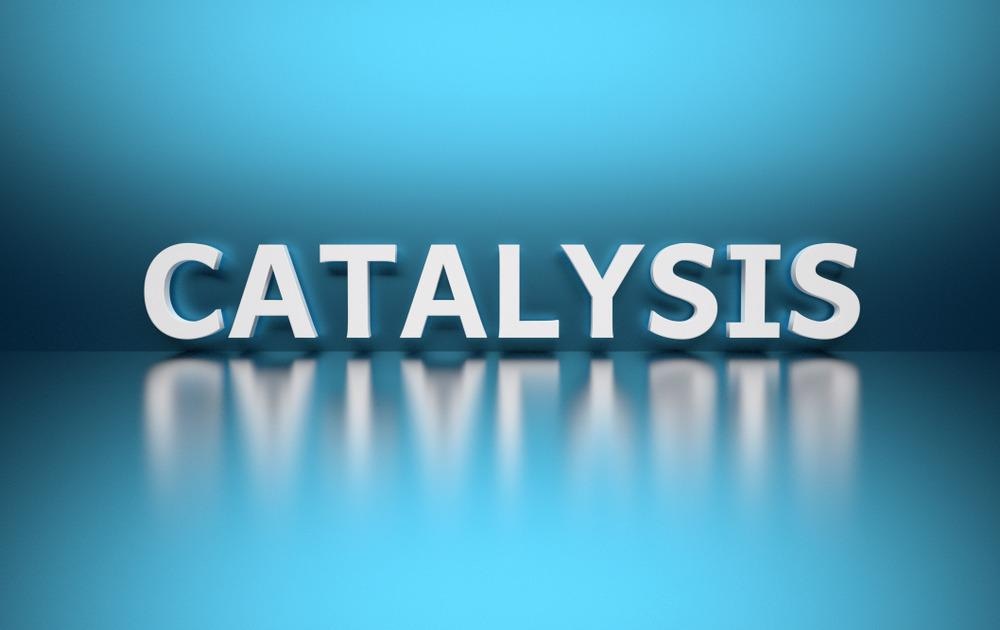
Image Credit: DariaRen/Shutterstock.com
Industrial catalytic reactors, where the reactant chemicals are exposed to a suitable catalyst, operate at elevated temperatures (often above 400 °C) and pressures (above 100 bar). Despite being thermodynamically spontaneous, many chemical reactions, such as ammonia synthesis, would not occur under ambient conditions.
The presence of the catalyst and the optimal conditions in the reactor allow the chemical reaction to proceed with high efficiency and yield. In the last century, catalysis played an irreplaceable role in industry allowing the production of fertilizers, fuels, pharmaceuticals, industrial chemicals, and plastics.
Current estimates show that 90% of all modern industrial chemical processes rely on heterogeneous catalysts (a catalytic process where the phase of the catalysts differs from that of the reactants or products).
How to Overcome the Limitations of Traditional Catalysis?
However, traditional catalysts have well-known limitations regarding their reactivity (limited by the available surface area of the catalyst), selectivity, and stability. Moreover, current environmental concerns drive the development of novel, more energy-efficient catalytic reactions that could be a significant step toward future carbon-neutral green chemistry.
Photocatalysis utilizes semiconductor materials as catalysts to convert light (including sunlight) into charge carriers (holes and electrons) that can promote chemical reactions. The energy absorption band of the photocatalyst and the redox potential of the charge carriers are controlled by the semiconductor bandgap.
The main limitations of a semiconductor-based photocatalytic process are the relatively low light absorption coefficient, the restricted wavelength absorbance range, and the low selectivity toward specific chemical reaction pathways.
Plasmon-Enhanced Optical Properties
Nanophotonics, which includes exploring the behavior of light at the nanoscale, enables scientists to overcome the limits of classical light-matter interactions usually encountered in conventional semiconductor-based technology. The merger of chemistry, nanotechnology, and quantum electrodynamics offers the possibility to manipulate and concentrate electromagnetic energy at the nanoscale.
When illuminated, nanometer-sized metal-dielectric interfaces, such as nanoparticles or nanostructured periodic surfaces, can generate volume and surface charge density oscillations called surface plasmons. Such nanostructured interfaces form a special type of low-dimensional optical metamaterials called plasmonic (or nanophotonic) structures. Depending on the geometry of the structure and the surrounding medium, the plasmon resonances may propagate across the flat metal-dielectric interfaces, or be localized as oscillating electrons inside metal nanoparticles.
Light Controls Catalytic Reactions
Recent research demonstrated that such plasmonic nanostructures could be used to control the selectivity and reaction yield in heterogeneous catalysis. As a proof of concept experiment, a research group at Stanford University led by Prof. Jennifer Dionne demonstrated a plasmonic antenna-reactor structure consisting of gold nanobars (serving as a plasmonic antenna) surrounded by a thin layer of SiO2 and crossed on top with palladium hydride nanorods.
Palladium can catalytically split hydrogen molecules on its surface. The dissociated hydrogen atoms exhibit very high solubility and diffusivity in palladium.
Exposing such nanophotonic structure to light led to the dehydrogenation of the palladium hydride nanoparticles accompanied by hydrogen release. The researchers at Stanford employed optically coupled environmental transmission electron microscopy to observe the phase transition of the individual catalytic nanoparticles with high temporal resolution under different illumination and environmental conditions.
Tracking Chemical Transformations on the Atomic Scale
The gold nanostructures underneath the palladium nanoparticles acted as an antenna, transmitting the incident light to the catalyst. In the absence of light, the dehydrogenation reaction was most intense at the tips of the nanorods.
When the nanophotonic assembly was illuminated, Prof. Dionne and her colleagues discovered that the catalytic activity could be precisely controlled by adjusting the illumination conditions and the location of the gold nanobars, resulting in an enhanced catalytic activity at different hot spots along the palladium hydride surface. The Stanford researchers regard their work as an important step toward optimizing catalysis on the atomic scale.
Using a similar approach, researchers at the University of Cambridge demonstrated novel photoactive colloidal nanomaterials consisting of a semiconductor substrate and plasmonic gold nanoparticles. The plasmon-induced effects successfully catalyzed the formation of radical species (molecules with an unpaired electron) and products of their dimerization, where two radicals form a reversible carbon-carbon bond. By controlling the illumination intensity, the researchers were able to switch on and off the redox reaction repeatedly.
The nanophotonics-based catalysis can also help scientists to understand how the reactive surface area of the catalyst can be maximized. Such studies could be an impactful step toward more efficient catalysts, new types of catalytic transformations, and catalysts capable of mediating multiple chemical reactions simultaneously.
References and Further Reading
Huang, J., et al. (2021) Tracking interfacial single-molecule pH and binding dynamics via vibrational spectroscopy. Sci Adv., 7(23), eabg1790. Available at: https://doi.org/10.1126/sciadv.abg1790
Sokołowski, K., et al. (2021) Nanoparticle surfactants for kinetically arrested photoactive assemblies to track light-induced electron transfer. Nat. Nanotechnol., 16, 1121–1129. Available at: https://doi.org/10.1038/s41565-021-00949-6
Sytwu, K., et al. (2021) Driving energetically unfavorable dehydrogenation dynamics with plasmonics. Science, 371(6526), 280-283. https://doi.org/10.1126/science.abd2847
Disclaimer: The views expressed here are those of the author expressed in their private capacity and do not necessarily represent the views of AZoM.com Limited T/A AZoNetwork the owner and operator of this website. This disclaimer forms part of the Terms and conditions of use of this website.