Nanocrystals are crystalline particles with sizes in the range of 1 and 1000 nm and possess many unique properties. These nanoparticles are applied across many areas of science and engineering, including drug delivery, chemical catalysis, biological sensors, and optical devices. This article discusses the methods that can control the shape of nanocrystals, primarily to regulate nanoparticle complexity.
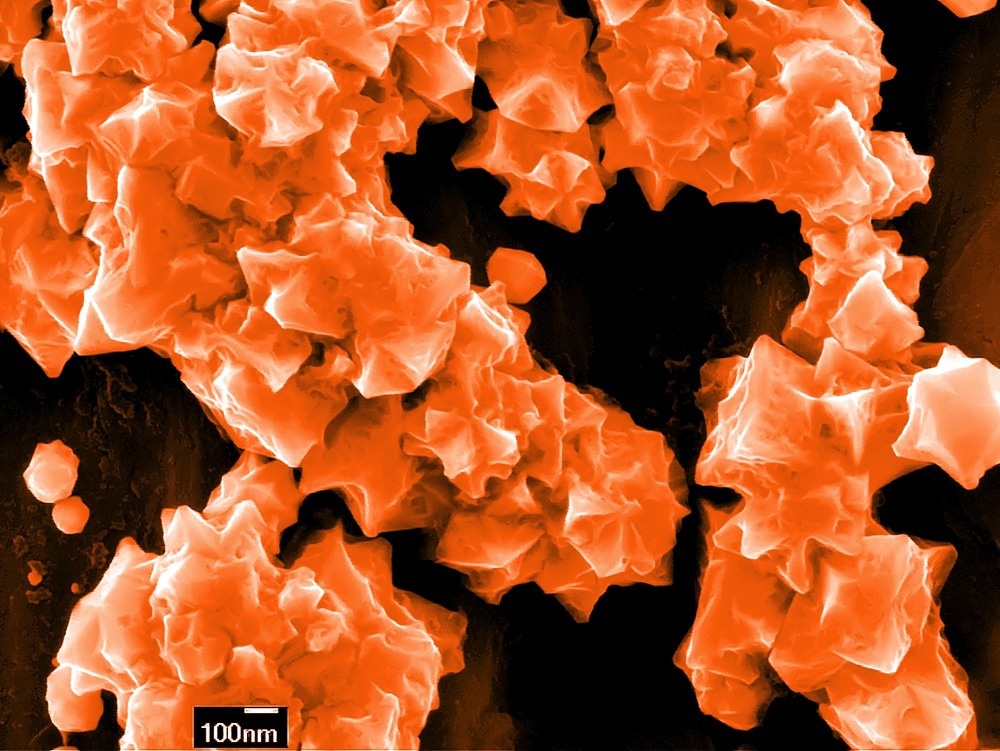
Image Credit: Georgy Shafeev/Shutterstock.com
Increased application of nanocrystals has emphasized the need to synthesize nanocrystals of specific shapes and sizes. There are different nanocrystal shapes, including spherical, rod, cubic, oval, triangular, hexagonal helical, dot, and prism. Nanocrystal shapes determine their properties, which are used for various applications. For instance, a nanoparticle with a lower surface edge and decreased coordination number exhibits superior chemical reactiveness, polarized surface, and greater catalytic activity.
Formation of Different Shaped Nanocrystals
Let us consider the development of zinc sulfide nanocrystal, which occurs via the diffusion of sulfur into a Zn nanocrystal suspension. This reaction results in the formation of building blocks that undergoes nucleation or self-assembly at specific nucleation centers to develop nanocrystals.
All the building blocks contain a specific number of atoms and the varied combination and types of interactions (chemical bonding, electrostatic attraction or repulsion, and mechanical stress).
The formation of a nanocrystal of a definite shape depends on the interaction between the building blocks in the self-assembling step. Therefore, by controlling the interactions between building blocks, the shape of nanocrystals can be regulated. Typically, scientists control the interactions between building blocks by using a mixture of surfactants and altering the reaction temperatures.
Chemical-Based Methods to Control the Shape of Nanocrystals
Chemical processes associated with the synthesis of nanocrystals involve two strategies, namely, co-precipitation and chemical reduction. Both the methods use a surfactant to control the growth process. Surfactants are molecules that contain a polar hydrophilic head and a hydrophobic hydrocarbon chain. A surfactant molecule with a small polar head and branched hydrocarbon chains form spherical water-in-oil droplets or reverse micelles.
Co-precipitation involves the thermal decomposition of organometallic precursors. The size of the newly synthesized nanoparticle is determined by the ratio of surfactant to precursor, and the shape of nanoparticles is controlled by the specific adsorption of the surfactant, which has been used to regulate the relative growth rates of crystal faces.
In the co-precipitation method, the reaction temperature and time play a vital role in synthesizing a particular nanocrystal. The chemical reduction method, occurring in colloidal assemblies, is another effective process for the size-and-shape-controlled synthesis of nanocrystals.
The shape of the nanoparticle depends on the shape of the template. For instance, if the reaction is carried out in spherical reverse micelles, the newly synthesized nanoparticles are mostly spherical. Although some exceptions to the above observation have been reported, in most cases, particularly for metallic (silver, gold, copper, etc.) and semiconductor nanoparticles (silver sulfide, cadmium sulfide, cadmium manganese sulfide, etc.), the above observation has been verified.
Scientists have successfully developed elongated copper nanocrystals by conducting the reaction in cylindrical reverse micelles or an interconnected cylinder phase. However, this process failed to perfectly deliver optimally shaped nanocrystals in every reaction.
To overcome this limitation, researchers have formulated a strategy by combining the surfactant-based template method and the capping method using salts or specific molecules. This method could control the shape of the newly synthesized nanoparticles. In certain instances, this method could also be used to obtain non-spherical shaped nanoparticles in spherical reverse micelles.
One of the defining factors associated with nanoparticle shape is their crystallographic characteristics related to the core and the enclosed surfaces. Hence, in a nutshell, the shape of a nanocrystal could be controlled via the structure of the precursor and the kinetics of particle growth could be regulated by the specific adsorption capacity of surfactant molecules or other molecules associated with the growing nanocrystal.
Over the years, scientists have determined factors influencing the formation of cubic nanocrystals. For instance, ionic selective adsorption is one of the main factors associated with the crystallographic nature of the surface and surface energies, which greatly vary according to the precursors used.
Mathematical Methods to Control the Shape of Nanocrystals
Besides chemical methods, many mathematical model-based software systems have been designed to predict the specific shape of a nanocrystal. Researchers have developed several mathematically distinct Wulff-based strategies to predict the shapes of nanocrystals. The modified Winterbottom model has been implemented in many commercial software packages to predict the shape of nanocrystals.
Hollow nanocrystals have gained popularity owing to their applications in developing lightweight materials. Additionally, these nanocrystals are also used in nanoelectronics, nano-optics, and drug delivery systems. Scientists manipulate the morphology of these nanostructures through mechanisms that are similar to the Kirkendall effect. Nanopores can be developed inside a nanocrystal through a mechanism of diffusion followed by nucleation.
A mathematical model has been used to attain the thermodynamic equilibrium shape of a nanocrystal based on surface energy minimization. This model was first formulated by Wulff in 1901. According to Wulff's construction, the equilibrium shape of single crystals could be determined based on a gamma plot (a plot of the orientation-dependent surface free energy). The modified model has been implemented for software development to visualize and quantify nanocrystal shapes.
References and Future Reading
Boukouvala, C., et al. (2021) Approaches to modelling the shape of nanocrystals. Nano Convergence, 8(26). https://doi.org/10.1186/s40580-021-00275-6
Liu, Z. et al. (2018) Chapter 2 - Shape control in the synthesis of colloidal semiconductor nanocrystals. Anisotropic Particle Assemblies, Synthesis, Assembly, Modeling, and Applications, pp. 37-54. https://doi.org/10.1016/B978-0-12-804069-0.00002-2
Tao, R. A. (2008) Shape Control of Colloidal Metal Nanocrystals, Nano Micro Small, 4(3). https://doi.org/10.1002/smll.200701295
Pal, S. et al. (2007) Controlling the Shape of Nanocrystals, Journal of Physical Chemistry C, 111(44), pp. 16071–16075. https://doi.org/10.1021/jp074950j
Lisiecki, I. (2005) Size, Shape, and Structural Control of Metallic Nanocrystals, Journal of Physical Chemistry B, 109, 25, pp. 12231–12244. https://doi.org/10.1021/jp058018p
Puntes, F.V. et al. (2001) Colloidal Nanocrystal Shape and Size Control: The Case of Cobalt, Science, 291(5511), pp. 2115-2117. DOI: 10.1126/science.1058495
Disclaimer: The views expressed here are those of the author expressed in their private capacity and do not necessarily represent the views of AZoM.com Limited T/A AZoNetwork the owner and operator of this website. This disclaimer forms part of the Terms and conditions of use of this website.