Raman spectroscopy has been highlighted as a vital tool for characterizing 2D materials-based van der Waal heterostructures. In this article, we explore its applications in analyzing graphene, TMDs and phospherene.
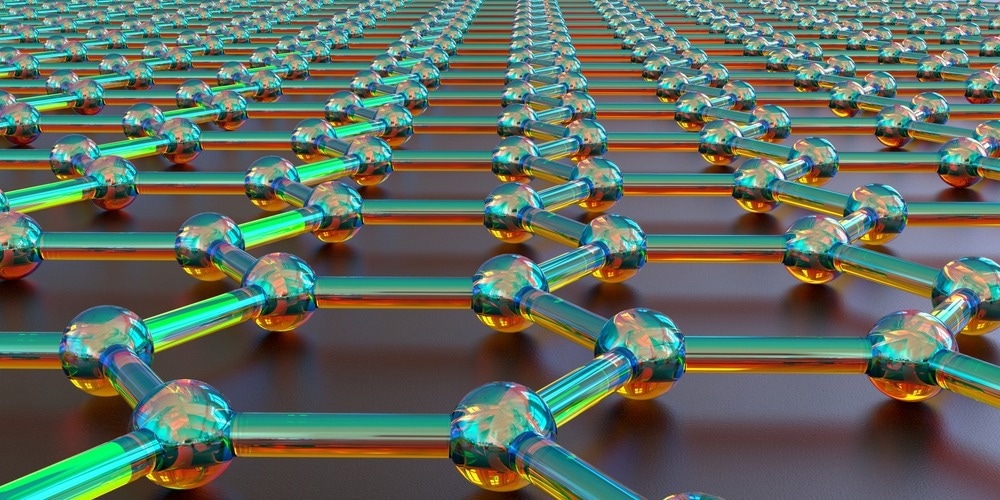
Image Credit: Kateryna Kon/Shutterstock.com
Two-dimensional (2D) materials are highly significant due to their extremely promising physical, chemical and mechanical properties. The discovery of graphene and the exploration of its attractive properties has triggered great interest in 2D materials.
Other than graphene, this category includes materials such as 2D silicene, phosphorene, hexagonal boron nitride, transition metal dichalcogenides, transition metal oxides and transition metal carbides/nitrides (MXenes). Their prominent quantum confinement has unleased exciting properties that cannot be found in other dimensional nanomaterials.
Raman spectroscopy is a powerful analytical tool for the characterization of the material to understand its crystallographic orientation. The vibrational modes of the molecules that are unique for specific molecules could be detected through Raman spectroscopy. The in-elastic scattering in the material upon the incident of the laser source is detected to identify the material and its nature.
Raman spectroscopy is a useful and non-destructive characterization tool to understand the physical and chemical properties of 2D materials.
How Does Raman Spectroscopy Work?
The light-matter interaction taking place in the material can be recorded in many ways. There could be several outcomes of the interaction depending on the nature of the light source and the properties of the material. A monochromatic laser source interacts with the molecule of the specimen material to scatter the light in the same energy, as of the incident photon, the phenomenon called Rayleigh scattering.
However, a small number of photons are scattered at a different frequency than the incident photon, called inelastic scattering. The phenomenon is also called the Raman effect, named after Sir C V Raman, who discovered this Nobel award-winning discovery in physics.
The vibration in the molecules upon light interaction creates a change in the electron cloud and, in turn, changes the polarizability of the molecule. These molecular bonds have specific energy transitions in which the polarizability change occurs, called the Raman active modes.
Raman spectroscopy instrumentation involves an excitation laser source, filters, detectors, sample holder and computer-compatible software for human interfacing. Laser sources with different wavelengths, ranging from ultraviolet (UV) through the visible to near-infrared (near- IR) could be chosen depending on the application.
Filters in Raman spectroscopy are used to separate the Raman scattered photons from the Rayleigh scattered ones. Notch, long pass and volume halogen filters are a few of the filters used to separate the inelastic scattered photons. These filtered photons then reach the detector, where the Raman signals are detected. Mostly, array detectors such as charged coupled devices (CCD) optimized for different wavelength sources are used to detect the Raman signals.
Raman Spectroscopy for 2D Materials
Raman spectroscopy conveys lots of information regarding 2D materials. Apart from the crystallographic configuration, information such as the number of layers, type of doping, strain and interlayer coupling could be analyzed through Raman spectroscopy.
2D Graphene Analysis Through Raman Spectroscopy
Defects in the 2D graphene layers could be detected by interpreting the obtained Raman signals. The G and 2D bands are the two Raman active modes obtained from graphene. The ratio of the intensity of these two peaks, ie. ID/IG, is usually utilized to understand the defect density in graphene layers, as reported in literature.
The interlayer interaction in the graphene significantly influences their properties. So, the number of layers of graphene could be determined through Raman spectroscopy. Raman 2D peak in graphene is utilized to discriminate between graphene and graphite, which are distinguishable by the number of layers.
2D graphene is a highly electrical/thermal conducting material with high mechanical strength and flexibility, most suitable for flexible devices with high performances. Raman spectroscopy is a useful tool for detecting material formation and quantitative analysis of the defect density of graphene.
Raman Spectroscopy for 2D Transition Metal Dichalcogenides
The three different polytypes in 2D transition metal dichalcogenides (TMDs), 2H, 1T and 3R, could be differentiated through the Raman active modes obtained from the respective materials. These three polytypes have different crystal structures due to different X-M-X stacking, where X stands for chalcogen and M for transition metal. There are mainly three Raman active modes in TMDCs; they are A1g, E1g and E12g, representing the out-of-plane relative motion of X atom, in-plane relative vibration of X atom and in-plane opposing motion of M-X atoms, respectively.
Many characteristic properties of the materials could be analyzed through the interpretation of these modes in TMDC. It was reported that the strain in TMDC was analyzed through the splitting of the in-plane vibrational Raman mode, E12g.
The mode was seen as sensitive external stimuli of strain and was seen splitting into two modes when the strain was applied. This splitting increased with the increase in the applied strain, as reported in literature.
The number of layers in 2D TMDCs could be determined through Raman spectroscopy. It is reported by Zhao et al. that the frequency E12g mode shows red shift, whereas a blue shift is seen in the frequency of A1g mode with the increase in the number of layers in the TMDCs.
The frequency difference between the A1g and E12g modes of the TMDCs is reported to identify the number of layers in TMDCs. Moreover, the full width at half maximum (FWHM) of these two modes was reported to decrease with the increasing thickness.
Many characteristic physical and chemical states of 2D TMDCs could be determined through Raman spectroscopy, which helps determine other properties. These materials have huge applications in optoelectronics devices due to their variable bandgap and high charge mobility.
Analyzing Phosphorene Through Raman Spectroscopy
The properties of another two-dimensional material, black phosphorous (BP), can be easily determined through Raman spectroscopy. In 2D monolayer of BP, called phosphorene, have a honeycomb-like structured arrangement of atoms. It is formed when a single phosphorous is connected to three other phosphorous atoms. A weak van der Waals force of attraction is found in between the layers of 2D BP in a stack.
As per reported by Zhu et al., there are mainly three Raman active modes observed in 2D BP: A1g, B2g and A2g. Amongst, A1g mode is reported to be affected by external conditions such as strain and thickness. On the other hand, A2g mode intensity was reported to determine the crystal orientation in BP.
Additional Applications of Raman Spectroscopy
Raman spectroscopy has huge applications in superconductivity, thermoelectricity, photoelectricity and many more. The determination of carrier concentration in semiconductors via doping or surface treatment could be easily determined through the shift in the Raman modes of the material.
In work by Chakraborthy et al., the effect of change in top-gate voltage on the carrier concentration in 2D MoS2-based transistors was determined by analyzing the shift of the A1g and E12g Raman active modes in the TMDCs.
Temperature-dependent Raman spectroscopy is an efficient tool to investigate the thermoelectric properties of 2D materials. It was reported by Zhang et al., that the A1g, B2g and A2g modes of phosphorene are more sensitive to temperature than graphene and MoS2 due to higher mechanical flexibility in few-layered BP.
Outlook on Raman Spectroscopy for 2D Materials Research
Raman spectroscopy is quite an effective research method for 2D materials. Many of the properties of 2D materials could be determined through this simple, non-destructive characterizing technique. It is an ideal tool for analyzing various interesting phenomena in the van der Waals heterostructure developed through different techniques.
Advances in this technique could drive an in-depth and accurate understanding of the various properties of these materials. This will further help in advancing the practical applications of 2D materials.
References and Further Reading
Liang, F., et al. (2018) Raman spectroscopy characterization of two-dimensional materials. Chinese Physics B, 27(3), p. 037802. doi.org/10.1088/1674-1056/27/3/037802.
Wu, J.B., et al. (2019) Raman spectroscopy of monolayer and multilayer graphenes. Raman Spectroscopy of Two-Dimensional Materials, pp. 1-27. doi.org/10.1007/978-981-13-1828-3_1.
Yin, P., et al. (2020) Applications of Raman spectroscopy in two-dimensional materials. Journal of Innovative Optical Health Sciences, 13(05), p. 2030010. doi.org/10.1142/S1793545820300104.
Zhao, W., et al. (2013) Lattice dynamics in mono-and few-layer sheets of WS 2 and WSe 2. Nanoscale, 5(20), pp. 9677-9683. doi.org/10.1039/C3NR03052K.
Zhu, W., et al. (2018) Anisotropic electron–phonon interactions in angle-resolved Raman study of strained black phosphorus. ACS nano, 12(12), pp. 12512-12522. doi/10.1021/acsnano.8b06940.
Chakraborty, B., et al. (2012). Symmetry-dependent phonon renormalization in monolayer MoS 2 transistor. Physical Review B, 85(16), p. 161403. doi.org/10.1103/PhysRevB.85.161403.
Zhang, S., et al. (2014) Extraordinary photoluminescence and strong temperature/angle-dependent Raman responses in few-layer phosphorene. ACS nano, 8(9), pp. 9590-9596. doi.org/10.1021/nn503893j
Disclaimer: The views expressed here are those of the author expressed in their private capacity and do not necessarily represent the views of AZoM.com Limited T/A AZoNetwork the owner and operator of this website. This disclaimer forms part of the Terms and conditions of use of this website.