In this article, we explore the benefits correlative microscopy can bring to nanoscience research. Continue reading to learn more.
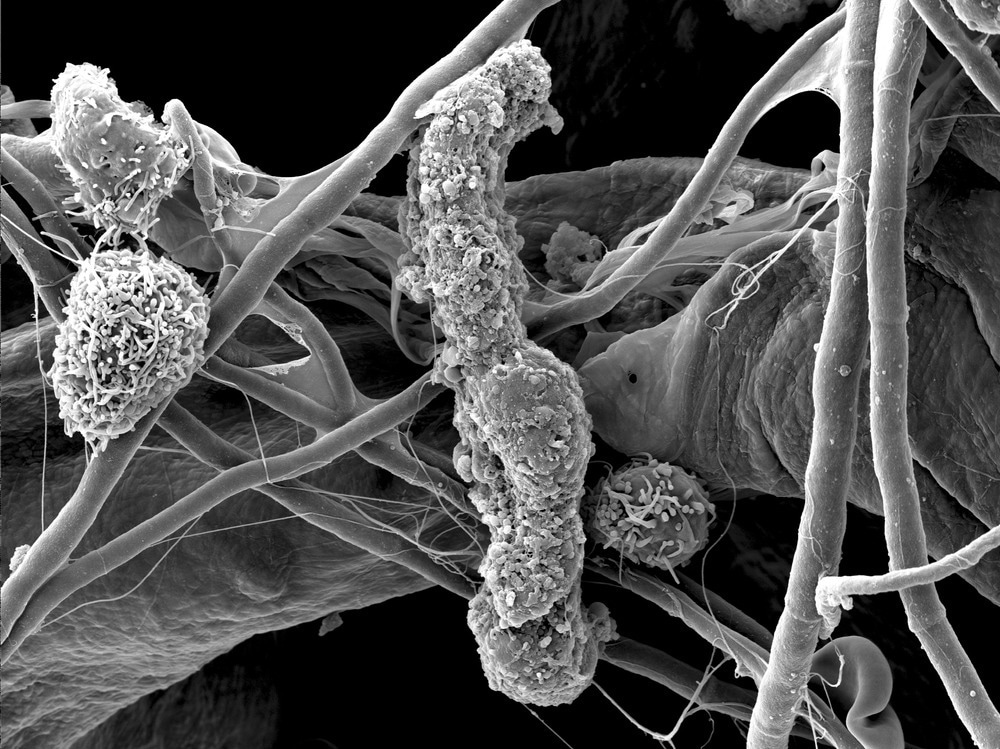
Image Credit: thomaslabriekl/Shutterstock.com
For nanotechnology and nanoscience applications, electron microscopy is one of the key characterization techniques for nanoanalysis.1 Few other imaging methods have the ability to resolve spatial features < 100 nm in size, and there are now a number of different electron microscopy approaches that can be used to recover information beyond simply identifying structural features, including elemental identification, quantification and three-dimensional imaging.2
One variation of electron microscopy is transmission electron microscopy (TEM). In a TEM experiment, the electron beam passes through the sample and the electrons are directly imaged onto an electron detector placed in a transmission geometry. The development of brighter electron guns and more sensitive detectors has been crucial in the development of TEM as a nanoscale analytical tool, as the samples used must be sufficiently thin for the electron beam to pass through, so the overall signal levels are very small.3
TEM generally offers the most powerful magnification of the electron microscopy techniques but has a few disadvantages in terms of the need for careful sample preparation and incompatibility with thicker samples.4 TEM generally uses higher incident electron energies and sample damage can also be a concern with many materials unable to withstand the intense electron beams.
As fabrication techniques have improved and nanoscale structures and devices have become more complex in terms of their design and functionality, nanoscience has grown in demand for more advanced imaging modalities that can provide greater information content and improved confidence over chemical and structural identification. So-called correlative microscopy approaches make use of a combination of different microscopy techniques on a single sample to provide complementary information that provides a more comprehensive characterization of the sample of interest.5
Correlative Microscopy
Correlative microscopy can use any type of microscopy approach, such as superresolution optical microscopy and electron microscopies, including TEM. As TEM is well-suited to mapping large image areas, and backscattered electrons can be collected simultaneously to provide elemental information, TEM is often combined with fluorescence microscopy approaches to perform what is known as correlative light electron microscopy (CLEM).6
A common issue in fluorescence microscopy is the need to find suitable fluorophores that interact with the biological species or functional site of interest. In CLEM, very different kinds of labels can be used, such as gold or platinum nanoparticles that interact with the site of interest and provide both strong fluorophores and are compatible as well with electron imaging.7
One advantage of CLEM is to improve the throughput of TEM methods. While TEM has an excellent spatial resolution, imaging large areas of a sample can be cumbersome and time-consuming. By locating the structures of interest with fluorescence microscopy first, only specific regions can then be imaged with the slower electron microscopy methods. If the fluorophores are highly selective and site-specific, fluorescence microscopy also provides additional identifying information, which may be difficult to retrieve from the electron microscopy images alone.
Applications
While there has been significant uptake of correlated microscopy methods involving electron microscopy in the life sciences community, particularly as cryogenic versions are now possible, CLEM methods have some useful applications for nanoscale materials studies.
Defect analysis is one particular application in materials science that has benefitted from correlative microscopy. Defects are small, localized sites in the lattice structure of a material where the regular pattern of the lattice is disrupted in some way.
A team of researchers made use of correlative TEM, 3D atom probe microscopy and contrast imaging techniques to study how defects and deformations formed in a CoNi-based superalloy.8
While heating the material, the team could probe in 3D the relative distances of atoms of the different elements and how they were affected through interactions with ion beams from different orientations and directions. As the nanoscale defects have a profound effect on the overall material properties of the sample, having methods sensitive to the structure and composition of the object that can be used to study the material under formation conditions is incredibly important for reliable manufacturing.
Outlook
Sample damage is a huge issue for correlative spectroscopy as the sample must be resistant to both damage from the intense electron beams and any other light sources used.9 Many fluorescence microscopy measurements make use of intensely focused laser sources, in particular femtosecond lasers with high peak powers, which can also cause problems with sample burning, and so the number of examples of correlative techniques where three or more methods are used are somewhat limited.
The use of cryogenic conditions can be beneficial, but improvements in detector sensitivity, reduced exposure times due to improvements in detection sensitivity and minimal scanning and sampling procedures will all reduce image acquisition times and, therefore, the radiation dose received by the sample.
Other new opportunities with correlative microscopy and TEM will come in the form of improved data analysis and evaluation of the multi-dimensional data acquired from the different techniques. More sophisticated correlation analysis will also help find new phenomena that correlative microscopy is uniquely sensitive to.
See More: How Advanced Microscopy Techniques Have Evolved Over Time
References and Further Reading
Wang, Z. L. (2003). New Developments in Transmission Electron Microscopy for Nanotechnology. Advanced Materials, 18, pp. 1497–1514. doi.org/10.1002/adma.200300384
Watanabe, M. (2013). Microscopy Hacks: Development of various techniques to assist quantitative nanoanalysis and advanced electron microscopy. Journal of Electron Microscopy, 62(2), pp. 217–241. doi.org/10.1093/jmicro/dfs085
Muller, D. A. (2009). Structure and bonding at the atomic scale by scanning transmission electron microscopy. Nature Materials, 8(4), pp. 263–270. doi.org/10.1038/nmat2380
Inkson, B. J. (2016). Scanning Electron Microscopy (SEM) and Transmission Electron Microscopy (TEM) for Materials Characterization. In Materials Characterization Using Nondestructive Evaluation (NDE) Methods. Elsevier Ltd. doi.org/10.1016/B978-0-08-100040-3.00002-X
Caplan, J., et al. (2011). The power of correlative microscopy: Multi-modal, multi-scale, multi-dimensional. Current Opinion in Structural Biology, 21(5), pp. 686–693. doi.org/10.1016/j.sbi.2011.06.010
Lange, F., et al. (2021). Correlative fluorescence microscopy, transmission electron microscopy and secondary ion mass spectrometry (CLEM-SIMS) for cellular imaging. PLoS ONE, 16(5 May 2021), pp. 1–17. doi.org/10.1371/journal.pone.0240768
Tanner, H., et al. (2021). Fluorescent platinum nanoclusters as correlative light electron microscopy probes. In Methods in Cell Biology (1st ed., Vol. 162). Elsevier Inc. doi.org/10.1016/bs.mcb.2020.12.002
Makineni, S. K., et al. (2018). Correlative Microscopy—Novel Methods and Their Applications to Explore 3D Chemistry and Structure of Nanoscale Lattice Defects: A Case Study in Superalloys. Jom, 70(9), pp. 1736–1743. doi.org/10.1007/s11837-018-2802-7
Crouzier, L., et al. (2021). Correlative analysis of the dimensional properties of bipyramidal titania nanoparticles by complementing electron microscopy with other methods. Nanomaterials, 11(12). doi.org/10.3390/nano11123359
Disclaimer: The views expressed here are those of the author expressed in their private capacity and do not necessarily represent the views of AZoM.com Limited T/A AZoNetwork the owner and operator of this website. This disclaimer forms part of the Terms and conditions of use of this website.