The development of nanorobots has significantly improved nanomedicine strategies. Although nanorobots serve as an excellent drug delivery system, fabricating magnetically controllable nanorobots with precise sizes, which are appropriate for drug delivery, has been a challenging task.
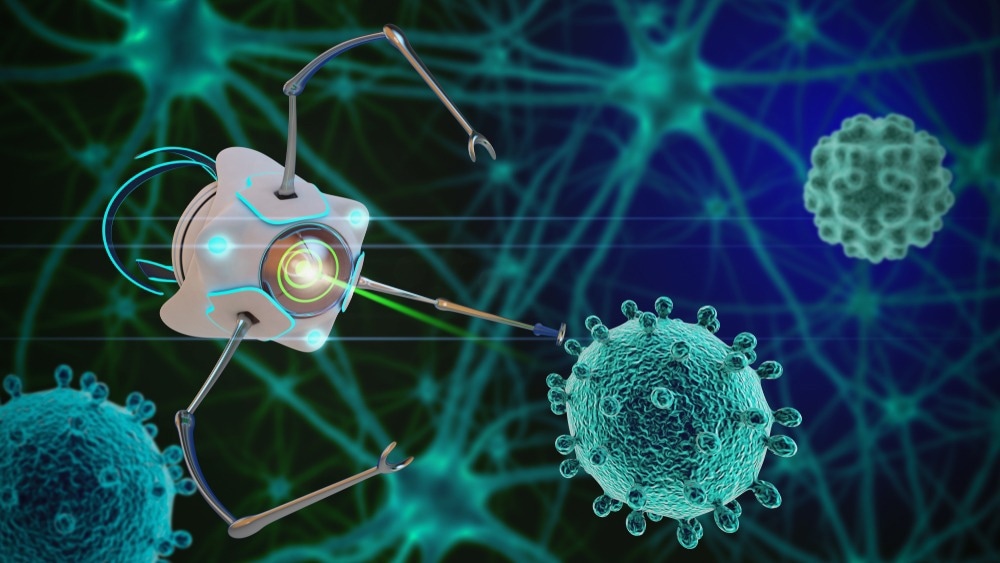
Image Credit: Volodymyr Horbovyy/Shutterstock.com
Recently, scientists have addressed this limitation and fabricated nanorobots using electron beam lithography, which is biocompatible with immune cells. This study has been published in Scientific Reports.
Conventional Methods to Create Nanorobots
Nanorobots are small-sized nano-based devices that can navigate distal regions of the body that cannot be easily reached. These devices have various biomedical applications, for example, minimally invasive surgery, remote sensing, and targeted drug delivery. Scientists have developed several micro/nanorobots with varied control methods, such as electric, acoustic, magnetic, thermal, and chemical.
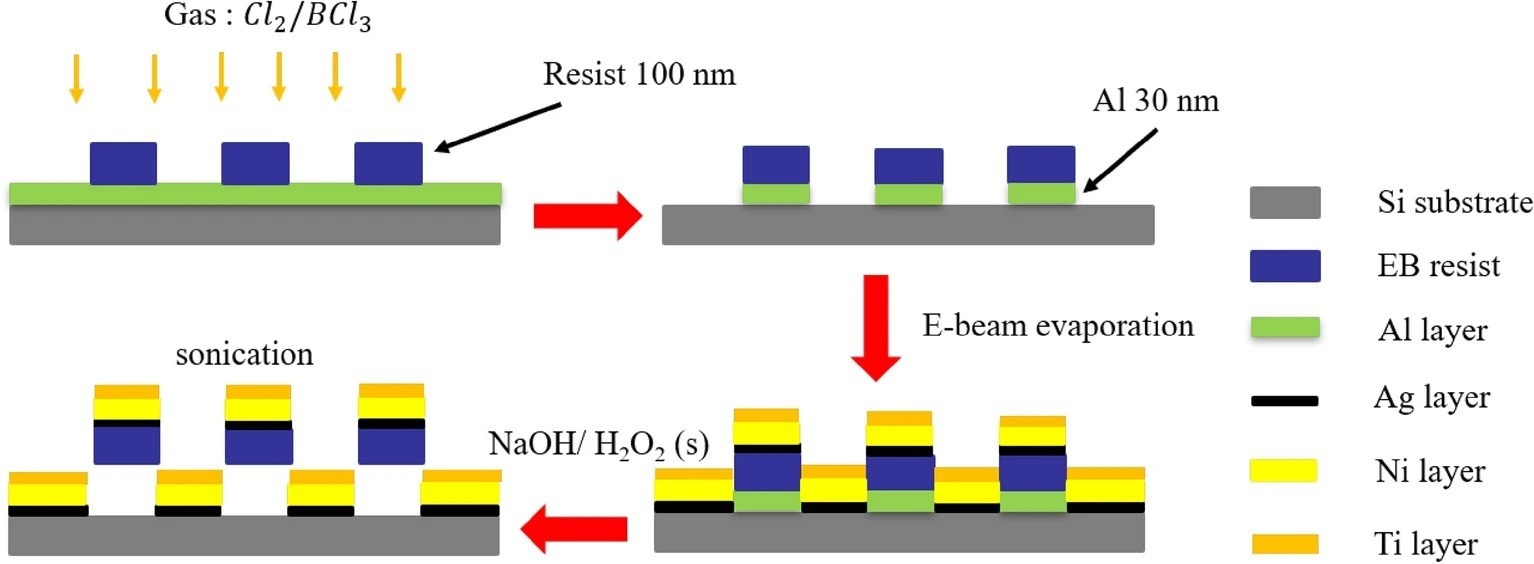
Figure 1. The preparation process of L-shaped nanorobots: (a) deposited Al layer through EBE, create resist patterns through EBL, and etch Al layer through ICP etching; (b) removed Al from regions not shielded by EB resist patterns; (c) deposited Ag, Ni, and Ti through EBE; (d) dissolved Al layer using NaOH and H2O2 solution to release the nanorobots. © Jiang, T. et al. (2022)
Although magnetic nanorobots have been the most studied among nanorobots, their in vivo application has been associated with several issues, including poor biocompatibility, mobility, size, and targeting capacity. Additionally, scientists have been working on developing mass-manufacturable micro/nanorobots at an affordable cost.
One of the most common methods used to fabricate magnetic micro/nanorobots is the direct laser writing (DLW) method, based on two-photon polymerization (2PP) technology. This method is ideal for developing a microrobot of any desirable shape and 100 nm in length, with low Reynolds number propulsion. The main disadvantage of this method is its incapability to create nanorobots of a desirable size that are suitable for drug delivery.
Even though the above-stated limitation linked with the DLW method has been overcome by the glancing angle deposition (GLAD) method, it can only fabricate helical micro/nanorobots. Importantly, scientists used photolithography to develop achiral planar microrobots for drug delivery purposes. This method can be used for the mass production of microrobots at a low cost. Several studies have shown that achiral microrobots can swim at low Reynolds numbers under magnetic actuation and effectively compete with helical micro/nanorobots.
Development of Achiral Planar Nanorobots Using Electron Beam Lithography
Recently, researchers have created achiral planar nanorobots using the electron beam lithography (EBL) method. This is a novel method for nanorobot fabrication since the conventional EBL method was only used for two-dimensional nanostructures.
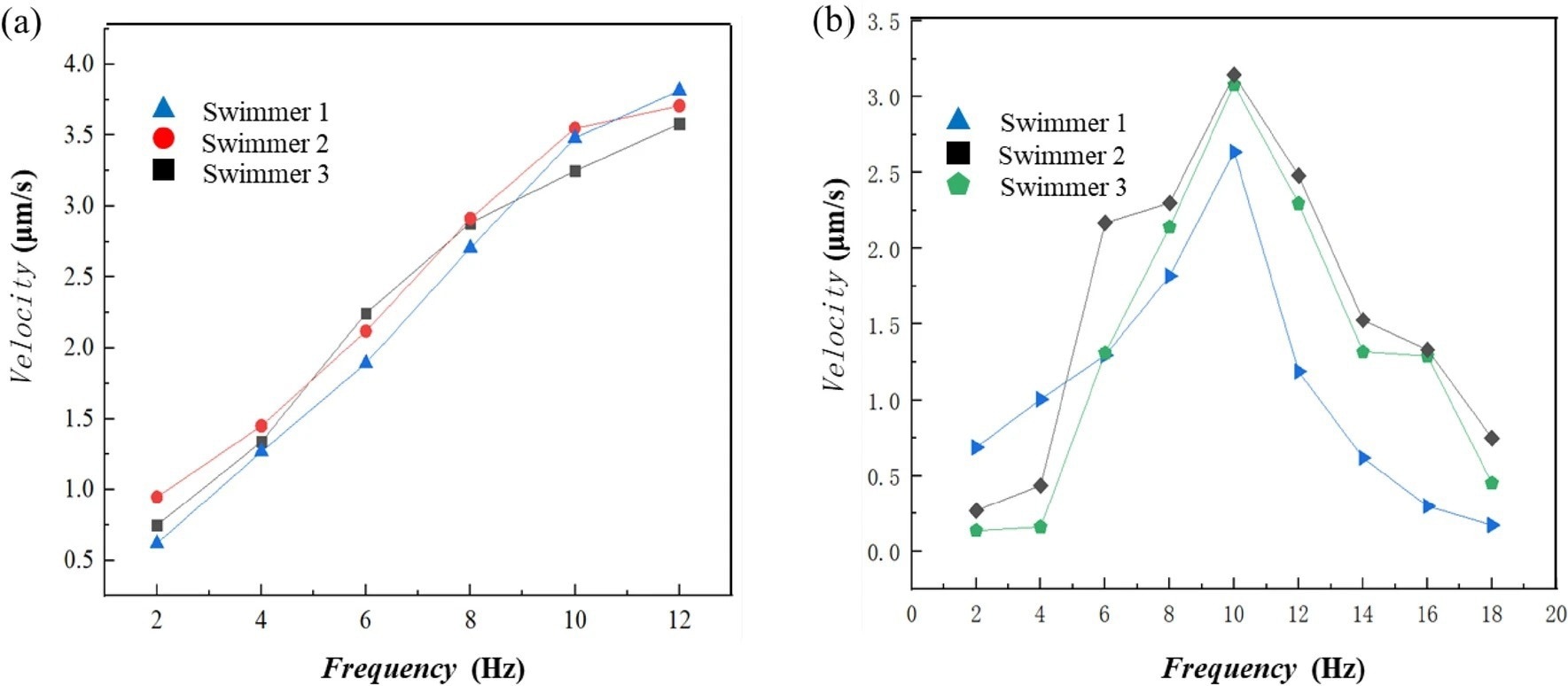
Figure 2. Velocity profiles of the nanorobots. (a) linear velocity profiles when the magnetic field frequency and strength increased proportionally. (b) nonlinear velocity profiles when the magnetic field strength remains constant at 2 mT while the frequency increases; step-out was observed at 10 Hz. © Jiang, T. et al. (2022)
In this study, scientists used two methods, i.e., electron beam exposure and electron beam evaporation methods, to fabricate a large number of nanorobots on a silicon wafer. The size of these nanorobots was around 400 nm. The authors used an adhesion layer using silver (Ag) between the nickel (Ni) layer and a layer of the electron beam (EB) resist.
The authors stated that the Ni layer endowed magnetic properties to the newly synthesized nanorobots, while the titanium (Ti) layer made it biocompatible. The metallic Ti gets easily oxidized and forms a titanium oxide (10nm) layer on the L-shaped nanorobots. These Ti-based nanoparticle-like structures make the nanorobot cytocompatible and enable cell adhesion.
In a nutshell, the newly synthesized L-shaped nanorobots, composed of resist/Ag/Ni/Ti, have been bound to the substrate made up of an aluminum (Al) sacrificial layer. Scientists designed the release process to ensure that minimal debris of Ag/Ni/Ti was left in the sample. This process involved the removal of the excess sacrificial Al during the nanorobot synthesizing process.
Advantageous Properties of the New Nanorobot
Researchers assessed the swimming properties of the newly synthesized L-shaped nanorobots (length 1.4 μm) by proportionally increasing the strength of the magnetic field and rotating frequency. They observed an increase in the forward speed of the nanorobot with the enhancement of magnetic field frequency.
The velocity of the nanorobots was also determined by maintaining the magnetic field strength at 2 mT, while increasing the rotating frequency. This test exhibited a change in the nanorobots’ body-fixed rotation axis when the rotating frequency was increased. This result indicated a non-linear relationship between frequency and speed.
The current study reported that the forward speed of the nanorobots increased until it reached a peak at 3.2 μm/s, where speed then decreased. An average swimming efficiency of 0.23 was reported comparable to the existing micro/nanorobots.
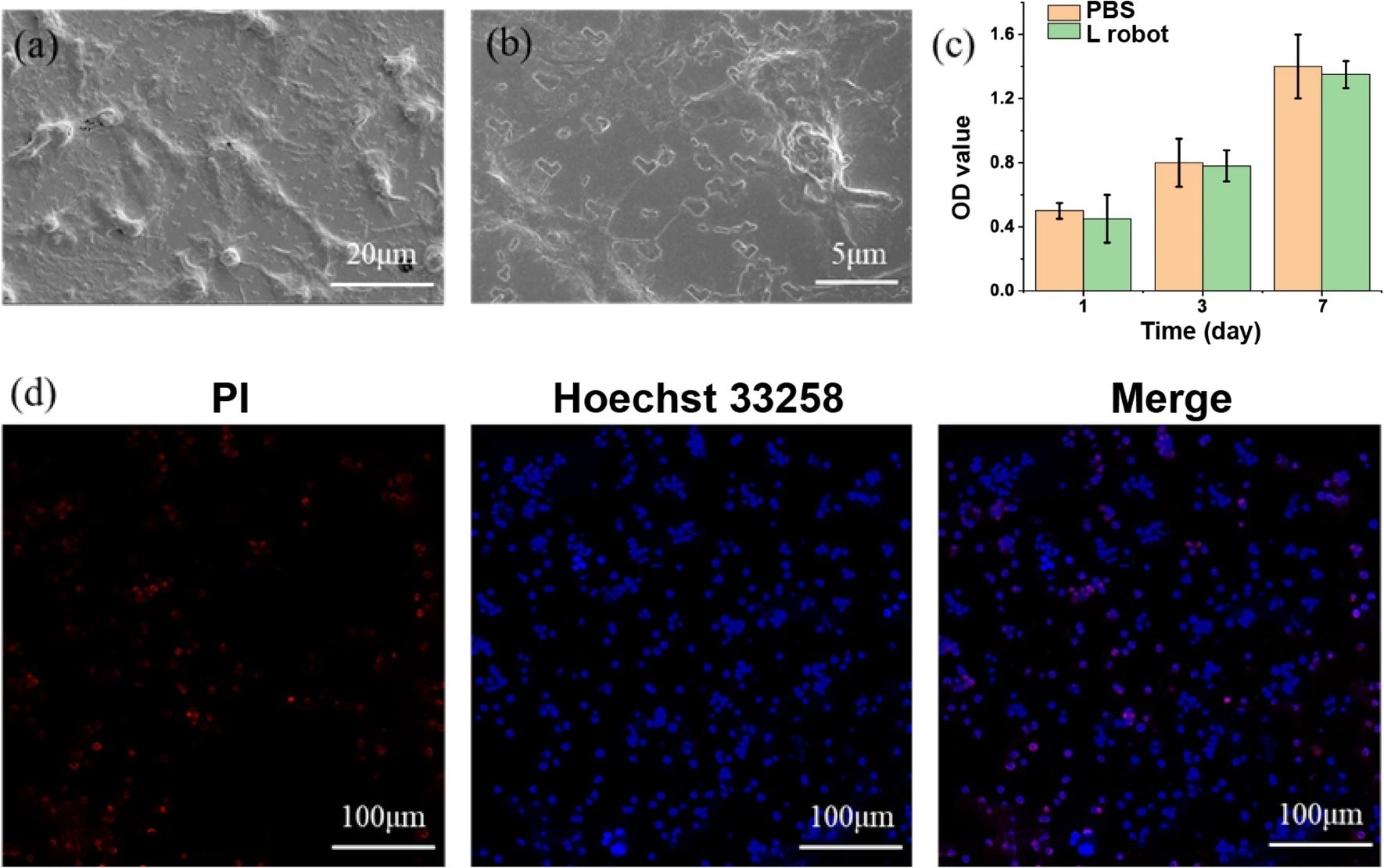
Figure 3. Cell cytotoxicity tests with HepG2 cancer cells. SEM image (a) and a zoom-in view (b) of L-shaped nanorobots cultured with HepG2 cancer cells. (c) Cell viability test using CCK-8 assay. (d) Live/dead by Hoechst 33258 (blue) /PI (red) staining. © Jiang, T. et al. (2022)
Biocompatibility of the L-Shaped Nanorobots for in vivo Applications
Scientists assessed the cytotoxicity of the L-shaped nanorobots via an CCK-8 assay, based on normal cells (Mouse fibroblasts L929) and cancer cells (HepG2 cells), to determine its in vivo applicability.
Scanning electron microscopy (SEM) analysis was performed to observe the adhesion of normal cells to the nanorobot. Scientists observed significant interfacial adhesion between the L-shaped nanorobots and cells. Additionally, the authors reported that the L-shaped nanorobots exhibited considerable proliferation compared to the control. This study also reported that the nanorobot did not show any cytotoxicity in HepG2 cells and normal cells.
Macrophages are crucial immune cells that play a critical role in tumor-targeted therapy and regenerative medicine. Hence, these immune cells were used to determine the cytocompatibility of the nanorobots. The authors reported that achiral nanorobots were stable and compatible with normal cells, cancer cells, and immune cells. Thereby, these L-shaped nanorobots have immense potential for in vivo nanomedicine applications.
Reference
Jiang, T. et al. (2022) Macrophage-compatible magnetic achiral nanorobots fabricated by electron beam lithography. Scientific Reports, 12 (13080). https://www.nature.com/articles/s41598-022-17053-x
Disclaimer: The views expressed here are those of the author expressed in their private capacity and do not necessarily represent the views of AZoM.com Limited T/A AZoNetwork the owner and operator of this website. This disclaimer forms part of the Terms and conditions of use of this website.