Although superconducting qubits have high prospects in quantum computers, existing qubit designs do not offer high-performance efficiency. An article published in Nature Communications presented “unimon” as a superconducting qubit type.
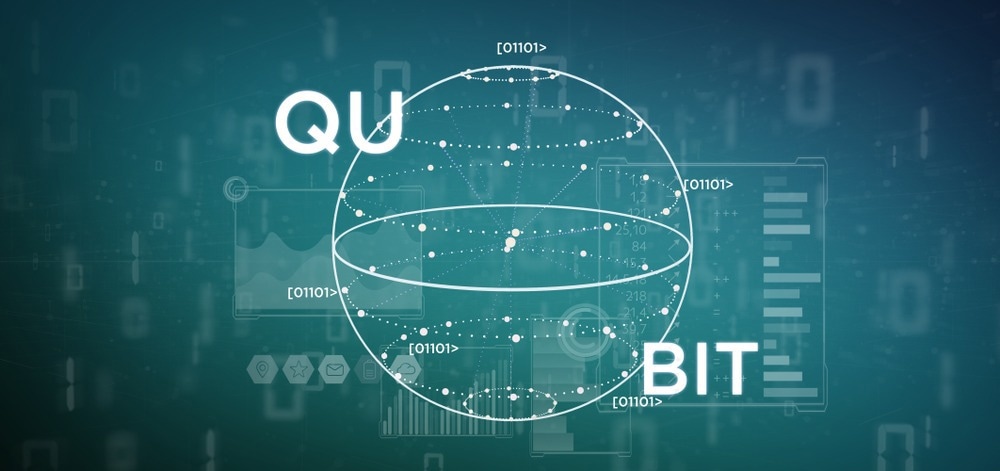
Study: Unimon qubit. Image Credit: Production Perig/Shutterstock.com
The fabricated unimon qubit exhibited desirable properties, including partial insensitivity to flux noise, complete insensitivity to noise, increased anharmonicity, and a simple resonator structure.
The qubit frequency and enhanced anharmonicity were measured at the optimal operating point, and a 99.9% fidelity was achieved for a gate duration of 13 nanoseconds. The domination of dielectric losses improved materials, designs, and gate time, resulting in 99.9% fidelity in the fabricated unimon for effective correction of quantum error.
Existing Qubits for Quantum Computers
Superconducting qubits have achieved quantum supremacy in some computations, but existing quantum computers still have defects owing to noise, which prevents them from being used in real-world applications.
The complexity of implementable quantum calculations in the noisy intermediate-scale quantum (NISQ) era is primarily restrained by defects in quantum gates with single and two qubits. In addition, to achieve nearly half of the time in a 100-qubit circuit, a minimum gate fidelity of 99.9% is required.
Moreover, the required fidelity for all quantum gates has not yet been demonstrated in any superconducting quantum computer because the number of qubits and, in particular, the gate depth required for a practical NISQ is considerable.
Transmon qubits, which can be reliably manufactured and have coherence times of up to several hundred microseconds, are currently used in most superconducting multiqubit processors, resulting in average gate fidelities of 99.8 to 99.9% for two-qubit gates and 99.98 to 99.99% for single-qubit gates.
By connecting a shunt capacitor in parallel to a Josephson junction and deriving the transmon from the charge qubit, it is possible to exponentially reduce the effect of charge noise on the transition frequency. However, owing to the large shunt capacitance, anharmonicity is limited to 200–300 megahertz.
Transmons can only implement quantum gates at a certain speed because leakage faults to states outside the computational domain must be suppressed. In addition, the transmon qubit readout speed is constrained by low anharmonicity and a strong readout tone excites the transmon to unconfined states outside the cosine potential. To speed up qubit operations and enable larger reliabilities within the bounds of the restricted coherence time, a higher anharmonicity is recommended.
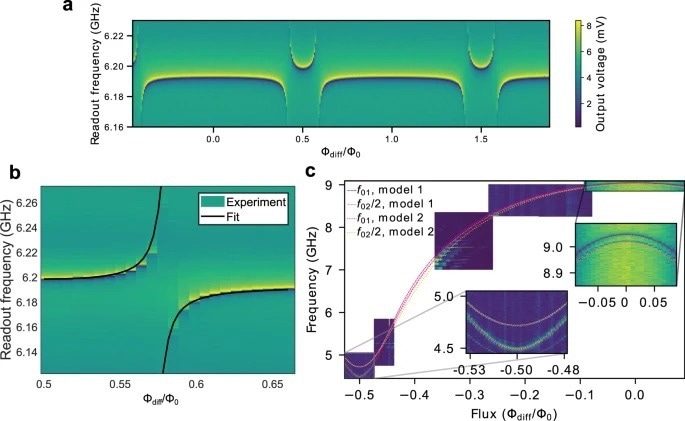
Figure 2. Resonator and qubit B spectroscopies. a Magnitude of the readout signal voltage transmitted through the probe line as a function of the signal frequency and the flux bias Φdiff of the unimon. b Magnification at an avoided crossings of a, where a unimon and its readout resonator are close to resonance, together with a fit (solid black line) used to estimate the coupling capacitance Cg between the qubit and the resonator. The fit is based on diagonalizing Eq (5) in “Methods”. c Magnitude of the readout signal at a properly chosen readout frequency as a function of the flux bias Φdiff and qubit excitation frequency, revealing the spectral lines of the unimon together with global fits to the theoretical model 1 and 2 (“Methods”). The insets show magnifications at the flux sweet spots, highlighting that at half flux quantum, the unimon frequency is minimized whereas its anharmonicity is maximized.
Unimon, A Novel Superconducting-Qubit Type
In this study, a chip consisting of three unimon qubits was fabricated, wherein niobium was used as the superconducting material in addition to the Josephson junction. Aluminum was used to fabricate superconducting leads.
In a recent press release, one of the authors of this study, Mikko Möttönen said, "Unimons are so simple and yet have many advantages over transmons. The fact that the very first unimon ever made worked this well gives plenty of room for optimization and major breakthroughs. As the next steps, we should optimize the design for even higher noise protection and demonstrate two-qubit gates".
The unimon qubit of this study was composed of a single Josephson junction shunted by a capacitor and a linear inductor via an unexplored method without the need for superinductor, resulting in high anharmonicity. Moreover, the designed unimon was partially shielded from flux noise and was resistant to low-frequency charge noise.
The primary advantage of the present work is that the fabricated unimon exhibits desirable properties, including reduced sensitivity to flux noise, complete insensitivity to direct current (dc) charge noise, increased anharmonicity, and a simple resonator structure in a single circuit.
Highlighting the significance of anharmonicity, one of the authors of this study, Eric Hyyppä said, "Because of the higher anharmonicity or nonlinearity, than in transmons, we can operate the unimon faster, leading to fewer errors per operation".
The frequency and anharmonicity measurements over a wide range of flux biases agreed with the first-principles models. The experimental data revealed that the dielectric loss affected the energy relaxation time.
Furthermore, the coherence time can be protected from noise at the flux-insensitive sweet spot. Notably, a decrease in gate duration resulted in an increased single-qubit gate fidelity of 99.9% for a 13 nanoseconds gate duration.
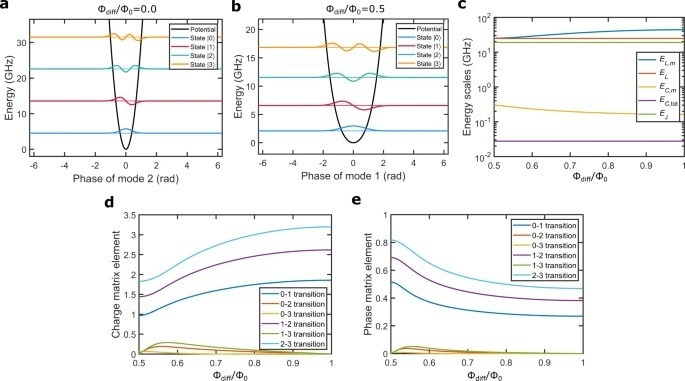
Figure 3. Potential-energy function, energy scales, and matrix elements of qubit B based on model 1. a, b Potential energy of the unimon based on equation (4) in Methods as a function of the phase variable φm of mode m together with the four lowest eigenenergies and corresponding phase-basis wave functions at flux biases of Φdiff = 0 (a) and Φdiff = Φ0/2 (b). Note that the second mode of the circuit is used as the qubit at Φdiff = 0 and the first mode at Φdiff = Φ0/2. c Energy scales EC,m, EL,m, EL, and EJ of the qubit as functions of Φdiff. Here, EC,m and EL,m are the capacitive and inductive energies of the qubit mode, EL is the inductive energy corresponding to a dc current in the center conductor of the qubit, and EJ is the Josephson energy. We also show an effective charging energy EC,tot = e2/(4Cll) computed based on the total capacitance 2Cll of the transmission line of the unimon. d Off-diagonal matrix elements |〈i|n^m|j〉||〈i|n^m|j〉| of the Cooper pair number operator n^mn^m for the four lowest-energy states of the qubit mode m as functions of Φdiff. e, As d but for the phase operator φ^mφ^m. In all panels, the results have been obtained by using the theoretical model 1 in “Methods” and the measured parameter values of qubit B reported in Table 1.
Conclusion
To conclude, this work, for the first time, demonstrated a unimon as a superconducting qubit. It was protected against both flux noise and low-frequency charge noise and had a relatively high anharmonicity while requiring a single Josephson junction and no superinductors.
Compared to junction-array-based superinductors in traditional fluxoniums, the geometric inductance of the unimon presented here offered enhanced predictability and reproducibility.
Additional improvements, such as reducing dielectric losses through improved design, utilizing low-loss materials, and reducing the gate duration to less than ten nanoseconds, can help develop a promising candidate for achieving single-qubit gate reliabilities with above 99.99% fidelity in superconducting qubits.
Future researchers anticipate minimizing on-chip cross-talk, scaling up to many-qubit processors, and implementing two-qubit gates. The researchers of the present study also suggested that it may be advantageous to decrease the footprint of a single unimon qubit by utilizing, for example, a superconductor with a high kinetic inductance in the coplanar-waveguide resonator to reduce the susceptibility of the unimon to flux noise and scale up the qubit count.
Reference
Hyyppä, E., et al. (2022) Unimon qubit. Nature Communications. https://doi.org/10.1038/s41467-022-34614-w.
Disclaimer: The views expressed here are those of the author expressed in their private capacity and do not necessarily represent the views of AZoM.com Limited T/A AZoNetwork the owner and operator of this website. This disclaimer forms part of the Terms and conditions of use of this website.