Agrotis ipsilon (Hufnagel) (Lepidoptera: Noctuidae) is a serious insect pest that damages many vital crops across the world. During their development, A. ipsilon larvae may eat up to 400 cm2 of vegetation. Chemical pesticides have been commonly used to prevent A. ipsilon from destroying crops.
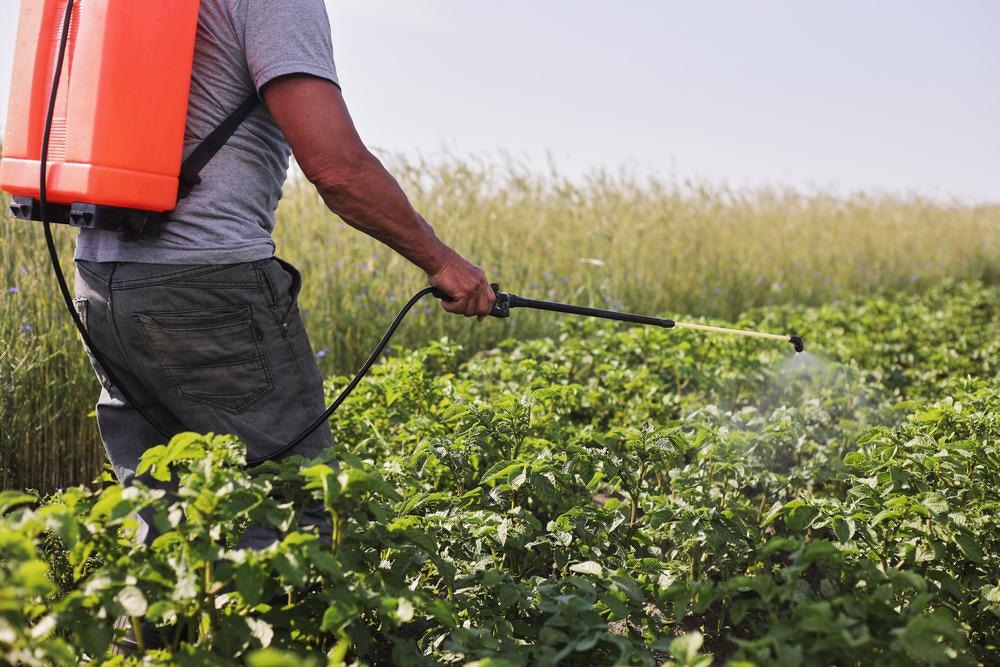
Image Credit: Andrii Medvediuk/Shutterstock.com
Nanotechnology may be able to provide alternative technologies to alleviate concerns regarding the negative environmental consequences of using chemical pesticides. Increased exposure and toxicity of these chemicals can affect non-target organisms and ecosystems. Nanoparticle technology might evolve in two ways: (a) as a single crop protection system, or (b) as pesticide carriers.
Insecticide research focuses on the effects of chemically active substances on insect growth and enzyme activities, both deadly and sublethal. Insecticides disrupt an insect’s functional balance (oxidative stress), by producing more reactive oxygen species (ROS) while impairing their scavenging mechanisms.
Insects have significant antioxidant enzymes such as superoxide dismutase (SOD), catalase (CAT), peroxidase (POX), and glutathione (L-glutamyl-L-cysteinylglycine, GSH). Superoxide dismutase (SOD) is an antioxidant enzyme that breaks down superoxide into oxygen and hydrogen peroxide. In all aerobic organisms, CAT is primarily an H2O2-scavenging enzyme that eliminates H2O2 produced by developmental or environmental stimuli into water and oxygen.
POX can oxidize a wide range of molecules with either H2O2 or O2, with H2O2 being used to oxidize phenolic compounds. GSH is an electron donor (cofactor) for antioxidant enzymes such as glutathione peroxidases and glutathione S-transferases. The inter-simple sequence repeats (ISSR) are a valuable marker for detecting genetic variation and distinguishing closely related people.
In new research published in the journal PLoS ONE, researchers investigated how A. ipsilon responded to nano-forms of two novel insecticides, chlorantraniliprole and thiocyclam. The study also investigated the effects of lethal and sublethal doses of both insecticides and their nano-forms on A. ipsilon development, reproduction, and oxidative stress enzyme activities including SOD, CAT, lipid peroxidase, and GR.
Methodology
In the laboratory, Agrotis ipsilon was maintained for 12 generations without pesticides in a rearing chamber. Researchers evaluated chlorantraniliprole and thiocyclam.
The finished nano-suspension was ready for TEM analysis. The toxicity of chlorantraniliprole, thiocyclam, and their nano-forms on second instar larvae was determined using a modified leaf dipping approach. On the second instar larvae, lethal dosages of chlorantraniliprole and thiocyclam were used to annihilate 15 and 50% of the larvae exposed to each form.
The total protein content of all samples was determined spectrophotometrically. Following the manufacturer’s instructions, DNA was extracted from both untreated and treated second instar larvae using a G-spinTM total DNA extraction kit.
Results
Top-down molecular chemical techniques were used to create nano-suspensions of chlorine in Figure 1 and Sulfur in Figure 2.
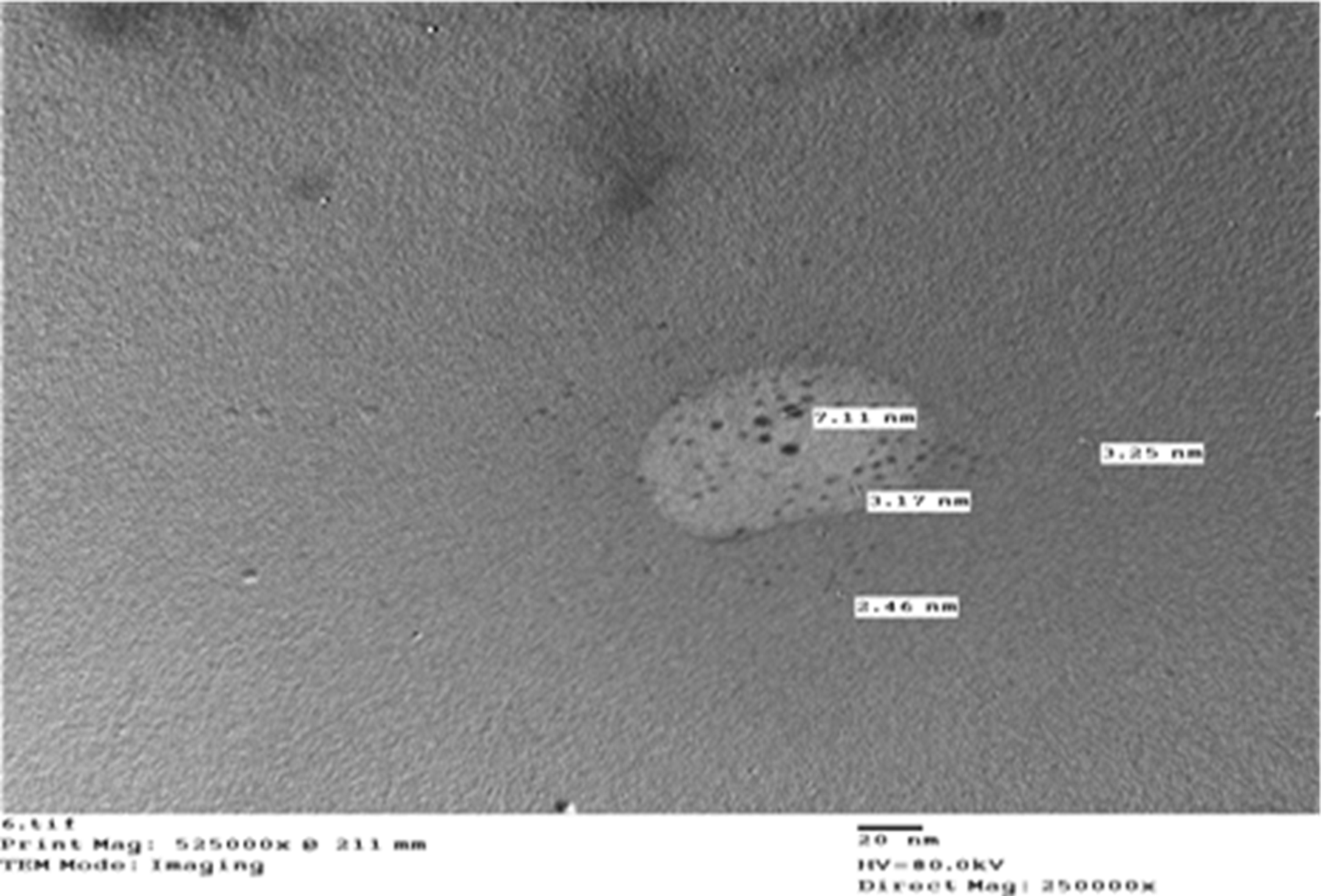
Figure 1. Nano-chlorine. Image Credit: Awad, et al., 2022
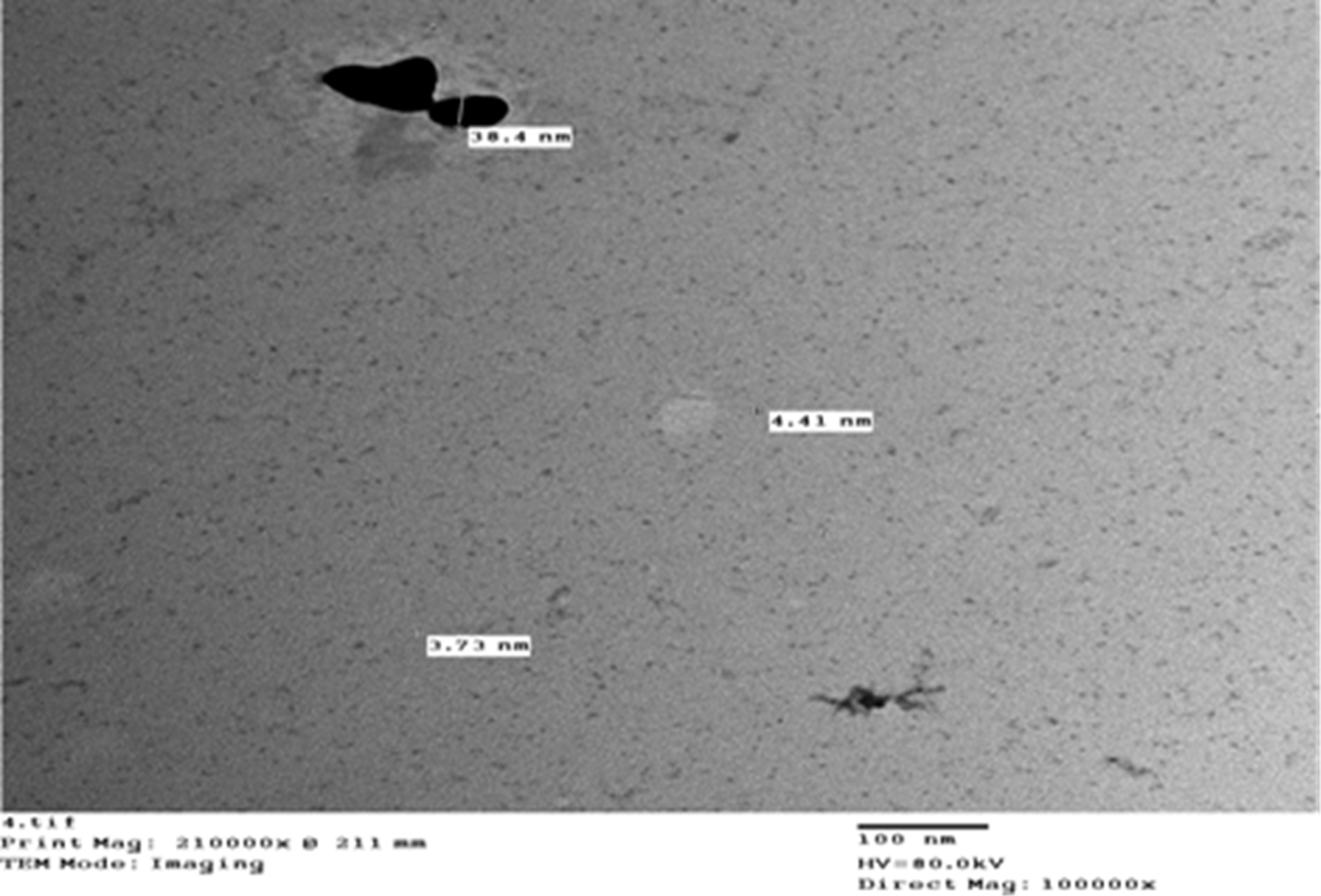
Figure 2. Nano-sulfur. Image Credit: Awad, et al., 2022
Table 1 shows the irregular form of the particles, with diameters of 4.05 nm for sulfur and 3.99 nm for chlorine, as well as 98.5% purity for each element.
Table 1. Particle size of nano-chlorine, and nano-sulfur. Source: Awad, et al., 2022
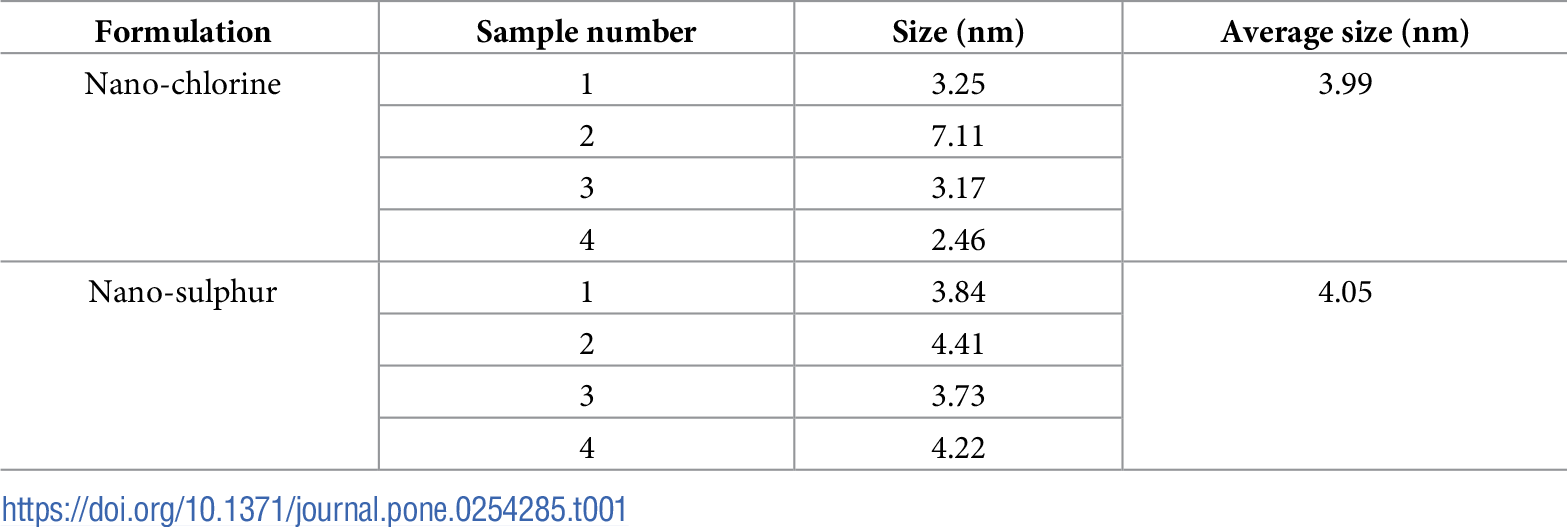
Table 2 displays the toxicity of chlorantraniliprole, thiocyclam, and their nano-forms on second instar larvae.
Table 2. Lethal and sublethal activity of chlorantraniliprole, thiocyclam, and their nano-form in the 2nd instar larvae of Agrotis ipsilon. Source: Awad, et al., 2022
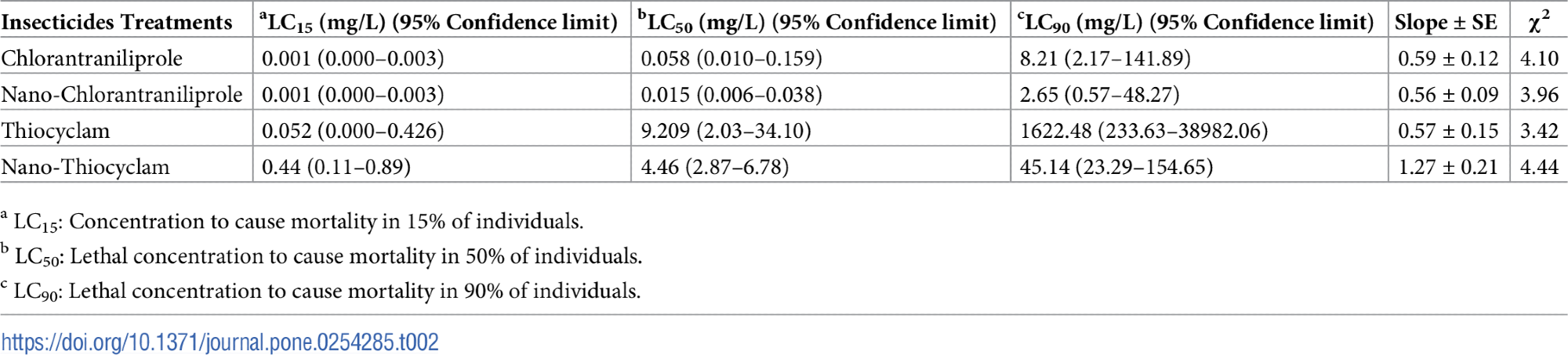
Table 3 indicates the latent impact of thiocyclam, chlorantraniliprole, and their nano-forms on A. ipsilon development owing to LC15 and LC50 exposure.
Table 3. Effects of chlorantraniliprole, thiocyclam, and their nano-form in the developmental stages of A. ipsilon. Source: Awad, et al., 2022
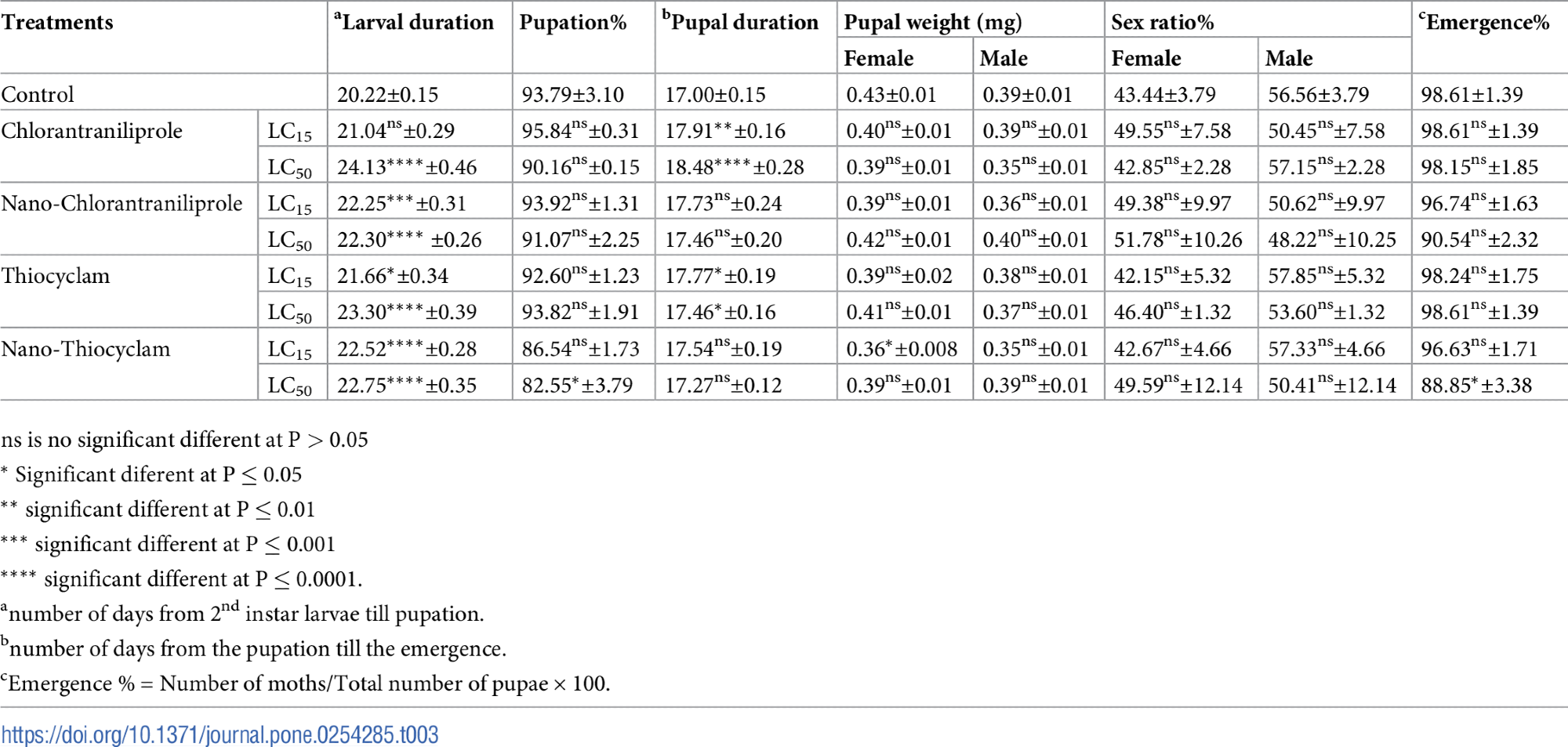
Compared to the control, chlorantraniliprole, thiocyclam, and their nano-forms drastically reduced hatchability % at LC15 and LC50 in Table 4.
Table 4. Mean fecundity and hatchability % (±SE) of A. ipsilon females after treating the 2nd instar larvae with LC15 and LC50 values of chlorantraniliprole, thiocyclam, and their nano-form. Source: Awad, et al., 2022
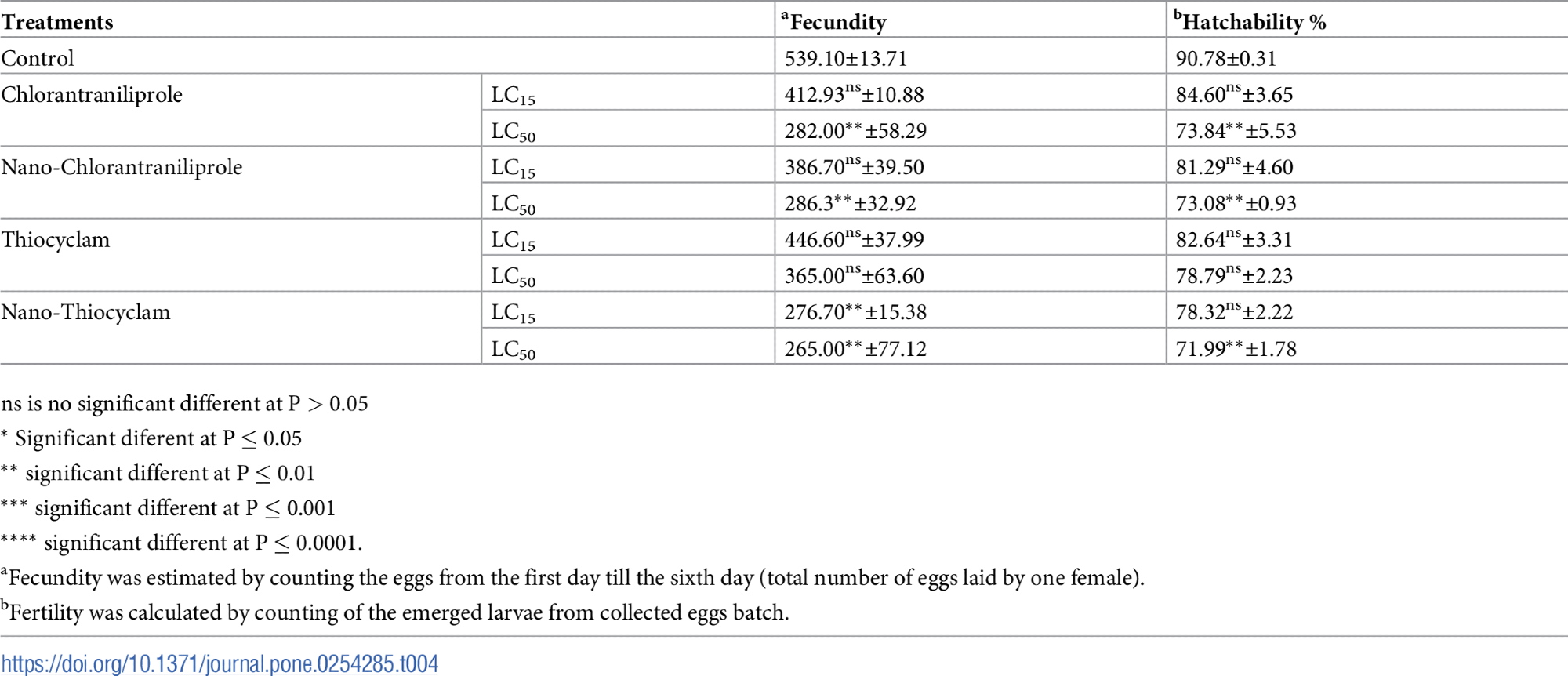
Following treatment with the LC50 of nano-thiocyclam (43.0 U/g of protein) and thiocyclam (40.09 U/g of protein), SOD activity increased significantly after exposure to both insecticides and their nano-forms as shown in Table 5.
Table 5. Mean (±SE) of oxidative stress enzymes (SOD, CAT, glutathione reductase, and lipid peroxidase) activities of A. ipsilon after exposure of 2nd instar larvae to LC15 and LC50 values of chlorantraniliprole, thiocyclam, and their nano-forms. Source: Awad, et al., 2022
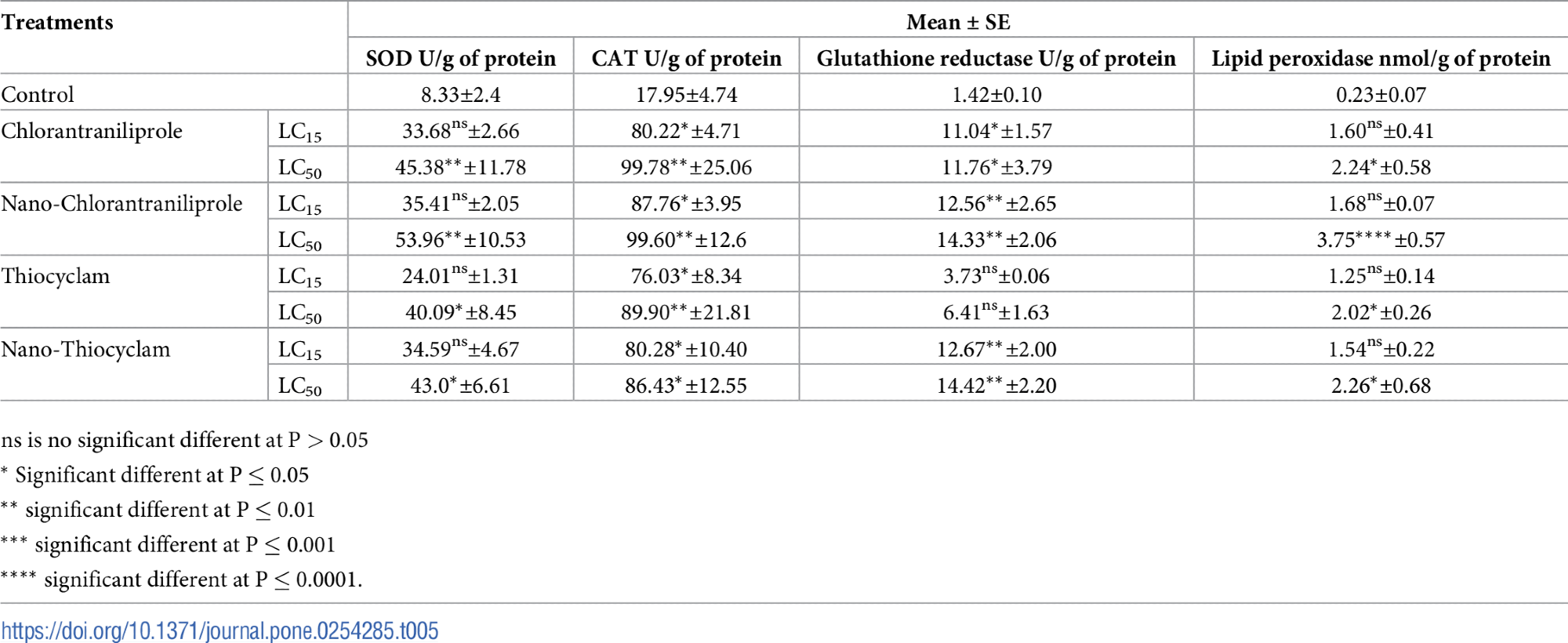
The silhouette analysis plots were created using the Euclidean distance metric to evaluate the cluster quality of the treatments on the basis of reproductive activity, enzymes, and developmental parameters using cluster distance tests within and between each cluster are depicted in Figure 3.
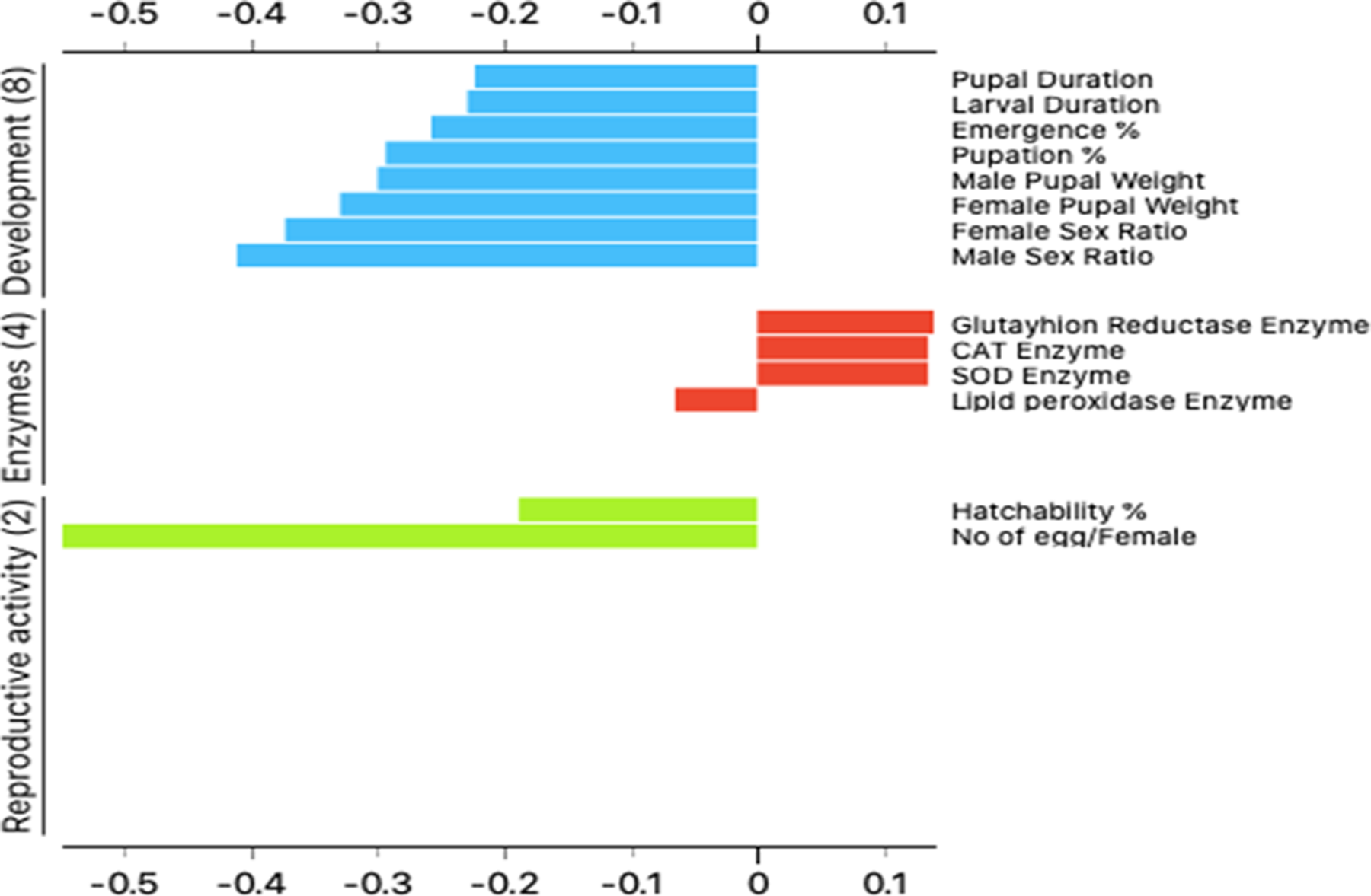
Figure 3. Plot of Silhouette analysis values for clustering of reproductive activity, enzymes, and developmental variables. On the y-axis each cluster are ordered by decreasing silhouette value. The silhouette value can range between −1 and 1. Image Credit: Awad, et al., 2022
The nano-thiocyclam LC15 was classified as less comparable to the control in the second cluster are seen in Figure 4.
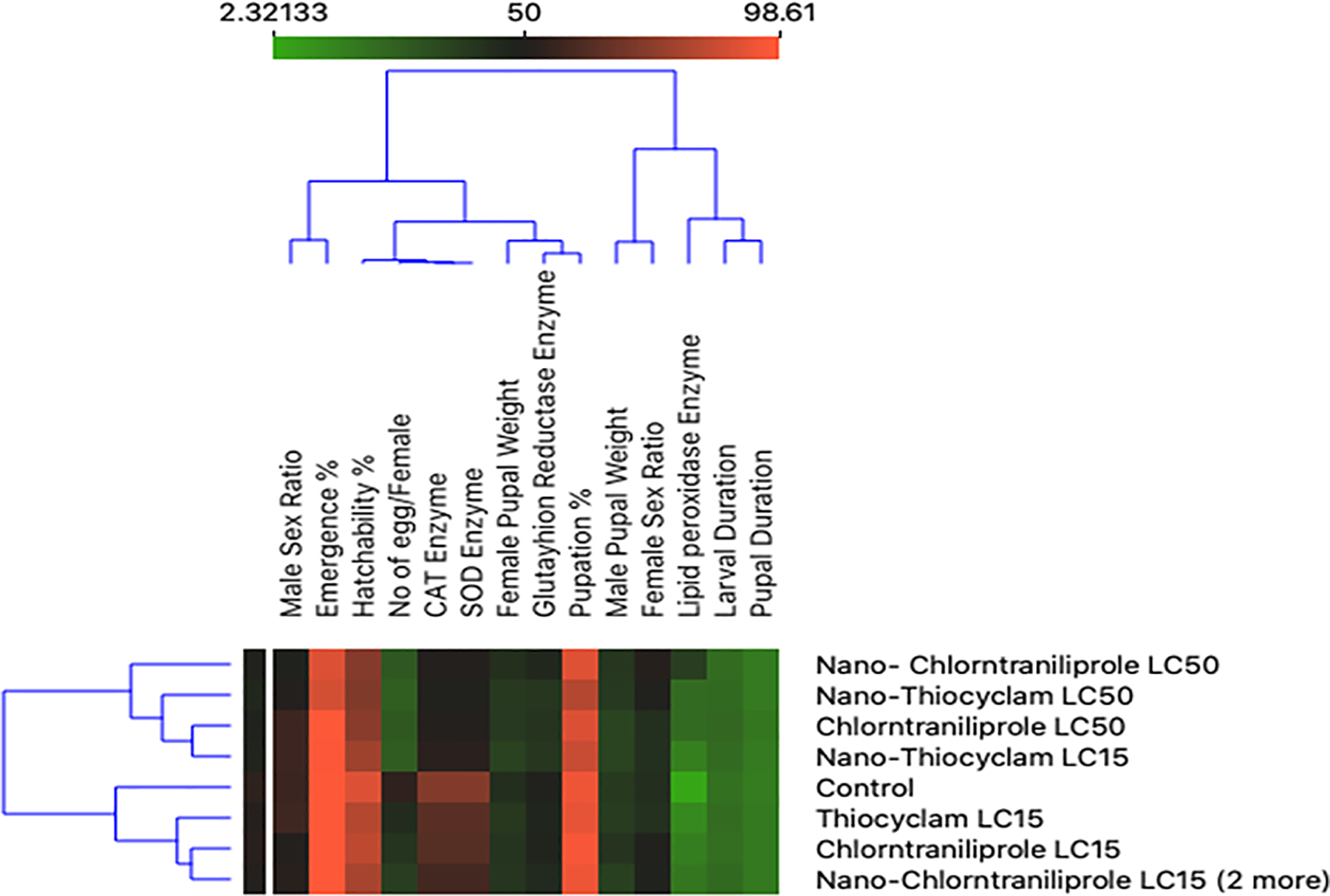
Figure 4. Two-dimensional heatmap visualization shows the interaction between the treatments and (A) the eight developmental parameters (B) the two reproductive activity parameters (C) the four enzymes’ parameters. Image Credit: Awad, et al., 2022
Figure 5 displays the effects of multidimensional preference analysis, which highlighted the interrelationships of all treatments, parameters, and classes.
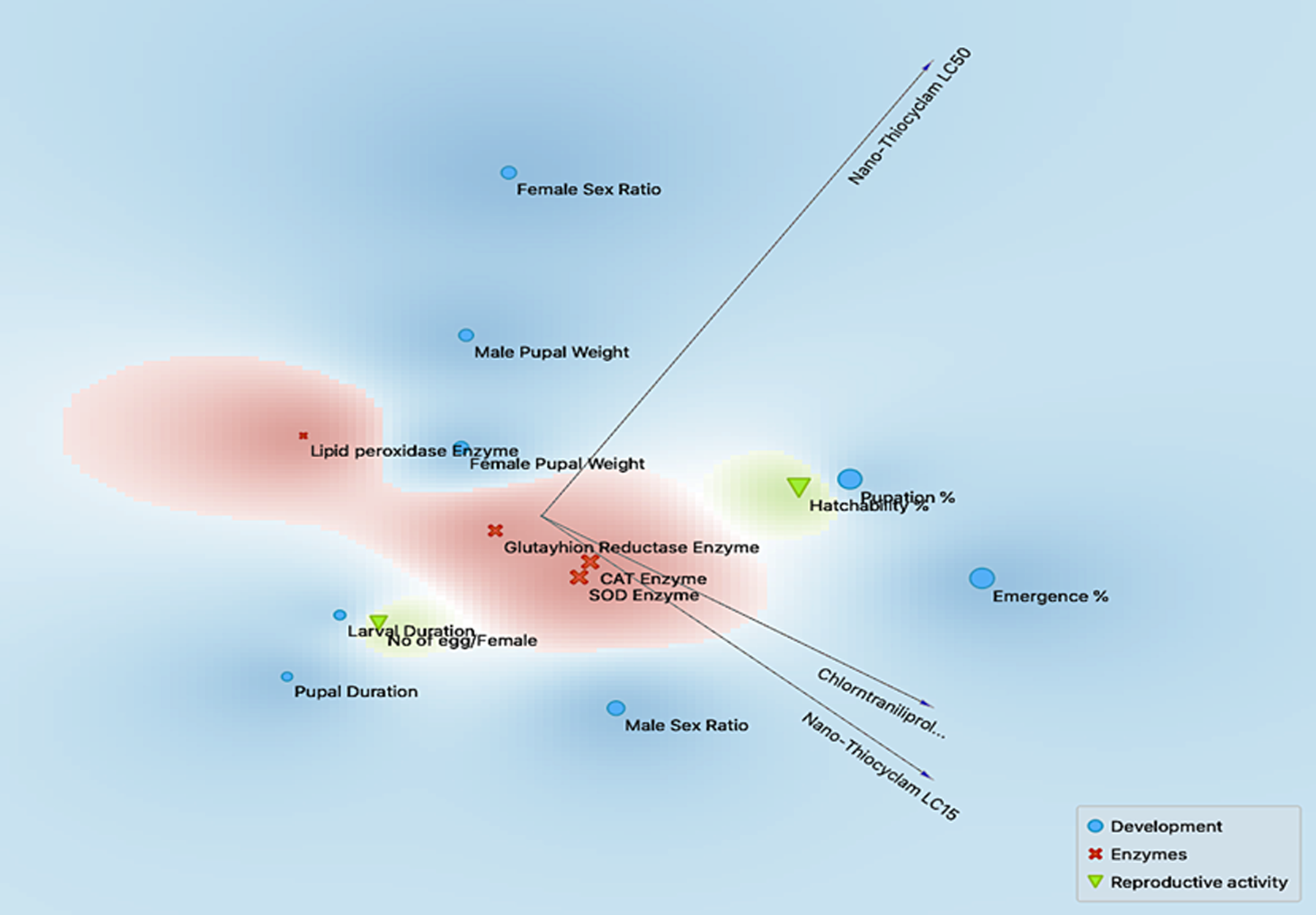
Figure 5. Multidimensional preference analysis plot summarizing the interrelationships amongst treatments, parameters, and classes. Image Credit: Awad, et al., 2022
15ISSR primers were used to amplify the DNA of the untreated second instar larvae and other treatments (Figure 6). With an average of 16.8 bands per primer, the 15 ISSR primers produced a total of 252 scoreable amplicons are displayed in Table 6.
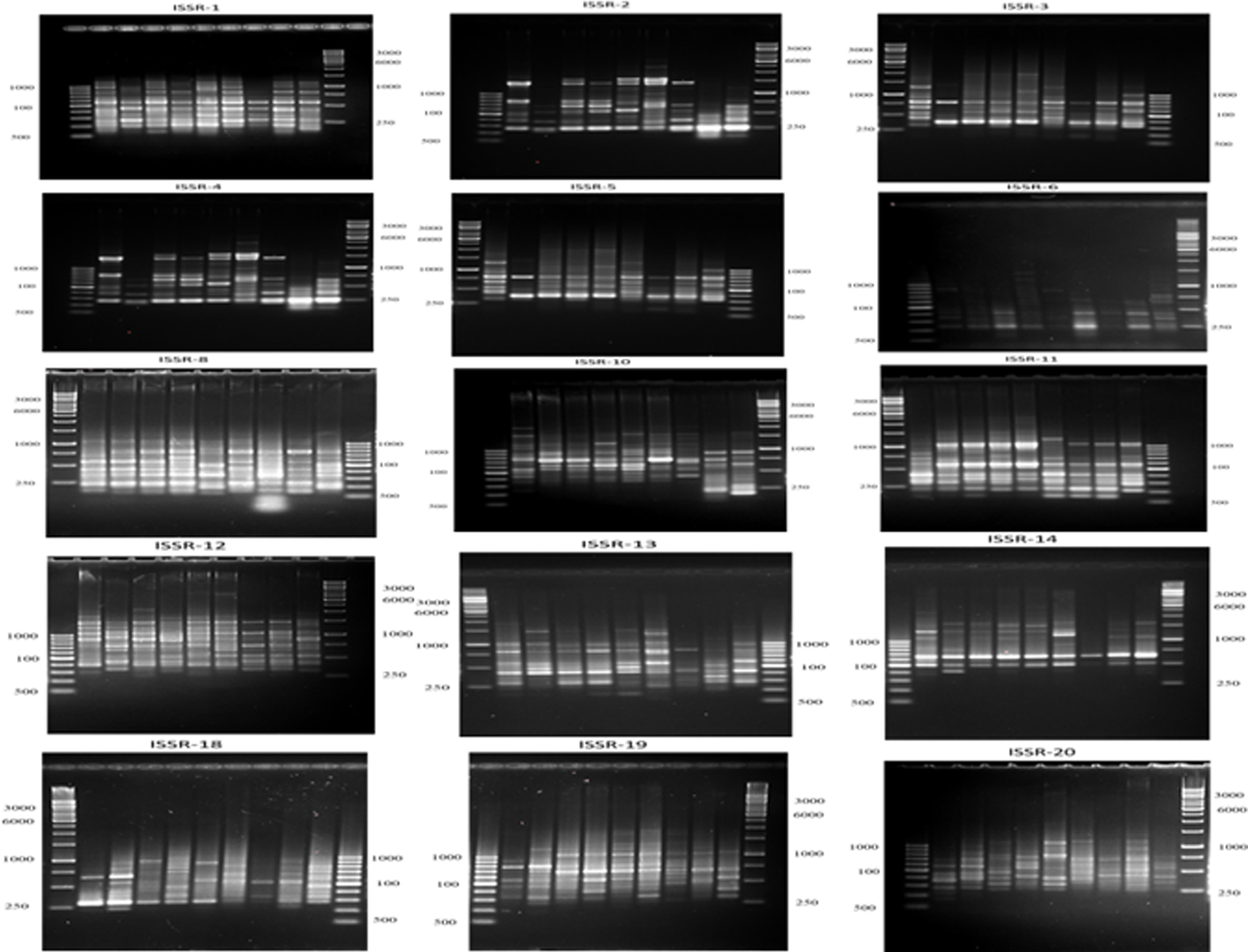
Figure 6. A representative agarose gel where PCR products of the 15 ISSR primers for the nine treatments. Image Credit: Awad, et al., 2022
Table 6. Primer names, number of total bands, polymorphic bands, percentage of polymorphism, and markers efficiency parameters of ISSR primers. Source: Awad, et al., 2022
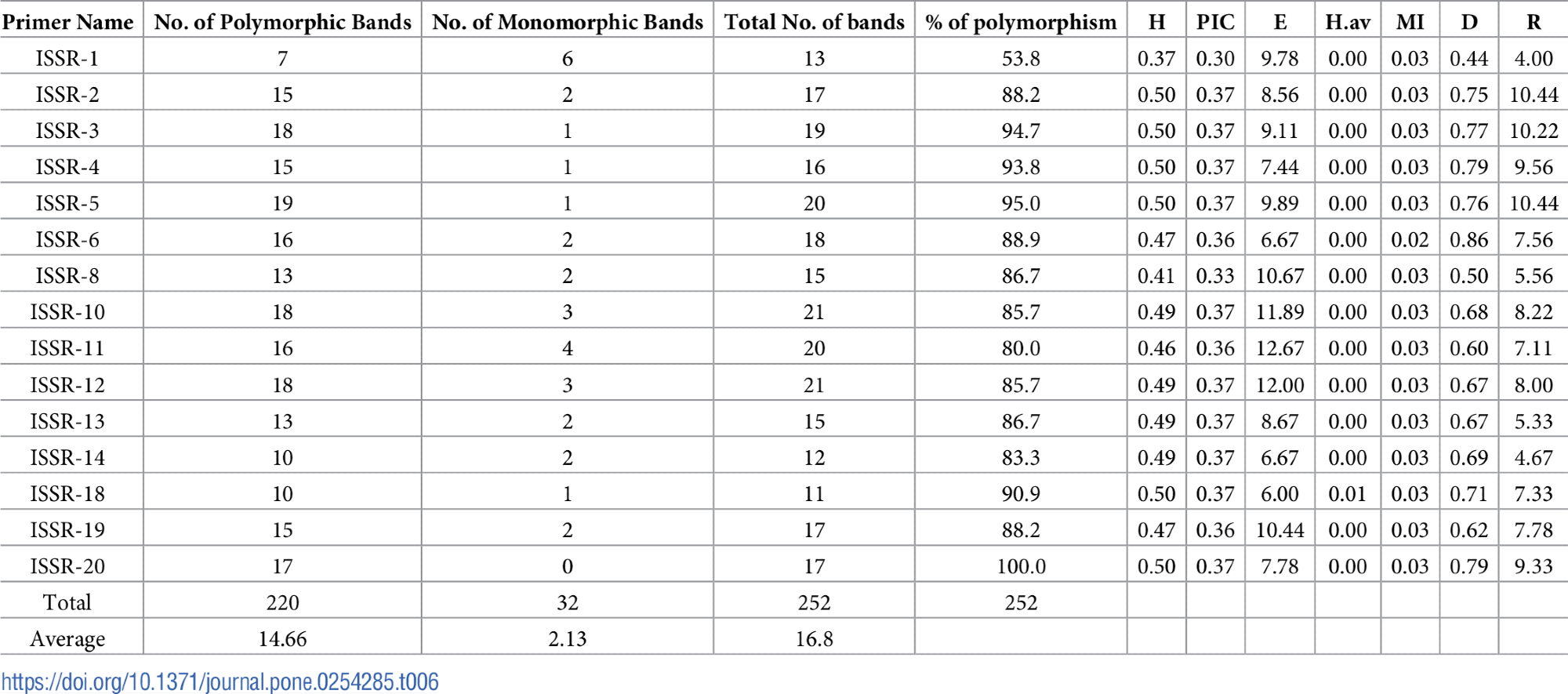
Table 7 shows the lowest GS, which was discovered to lie between the LC50 of nano-chlorantraniliprole and nano-thiocyclam.
Table 7. Genetic similarities between the nine treatments based on Jaccard’s similarity coefficient based on ISSR primers data. Source: Awad, et al., 2022
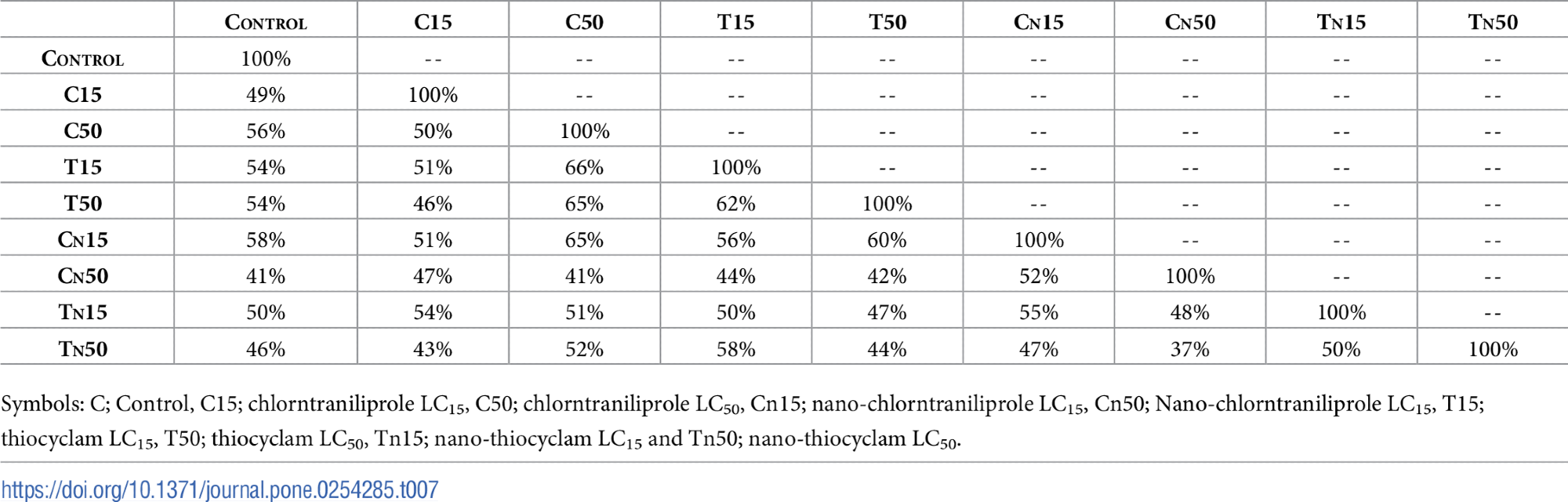
For the nine treatments, a dendrogram was created using UPGMA cluster analysis of the ISSR data is illustrated in Figure 7.
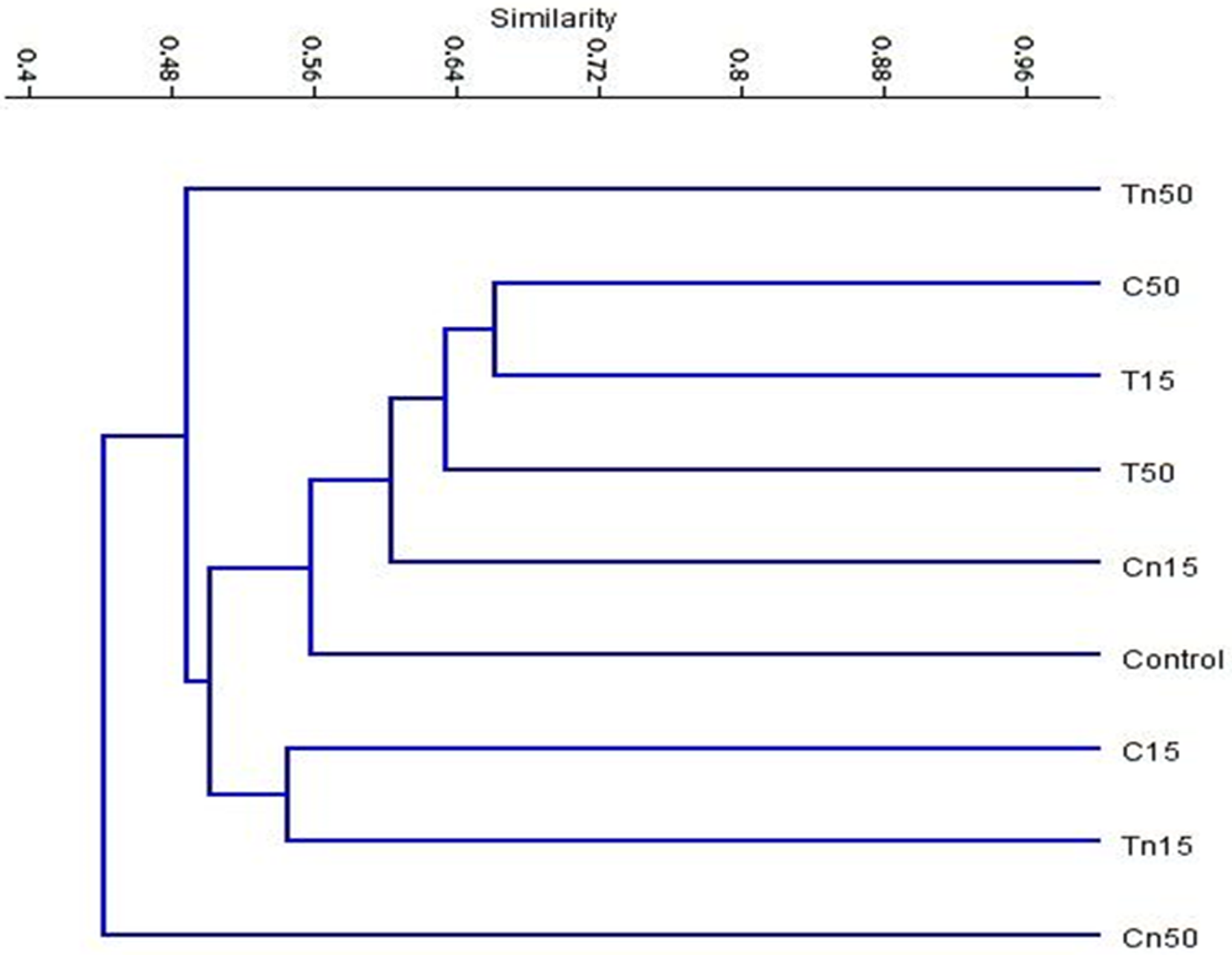
Figure 7. UPGMA cluster analysis based on Jaccard’s similarity coefficient of ISSR analysis of the nine treatments: C; Control, C15; chlorntraniliprole LC15, C50; chlorntraniliprole LC50, Cn15; nano-chlorntraniliprole LC15, Cn50; nano-chlorntraniliprole LC50, T15; thiocyclam LC15, T50; thiocyclam LC50, Tn15; nano-thiocyclam LC15 and Tn50; nano-thiocyclam LC50. Image Credit: Awad, et al., 2022
Figure 8 shows the PCA findings that showed the LC15 of nano-chlorantraniliprole and the LC50 of thiocyclam were the most comparable to the control.
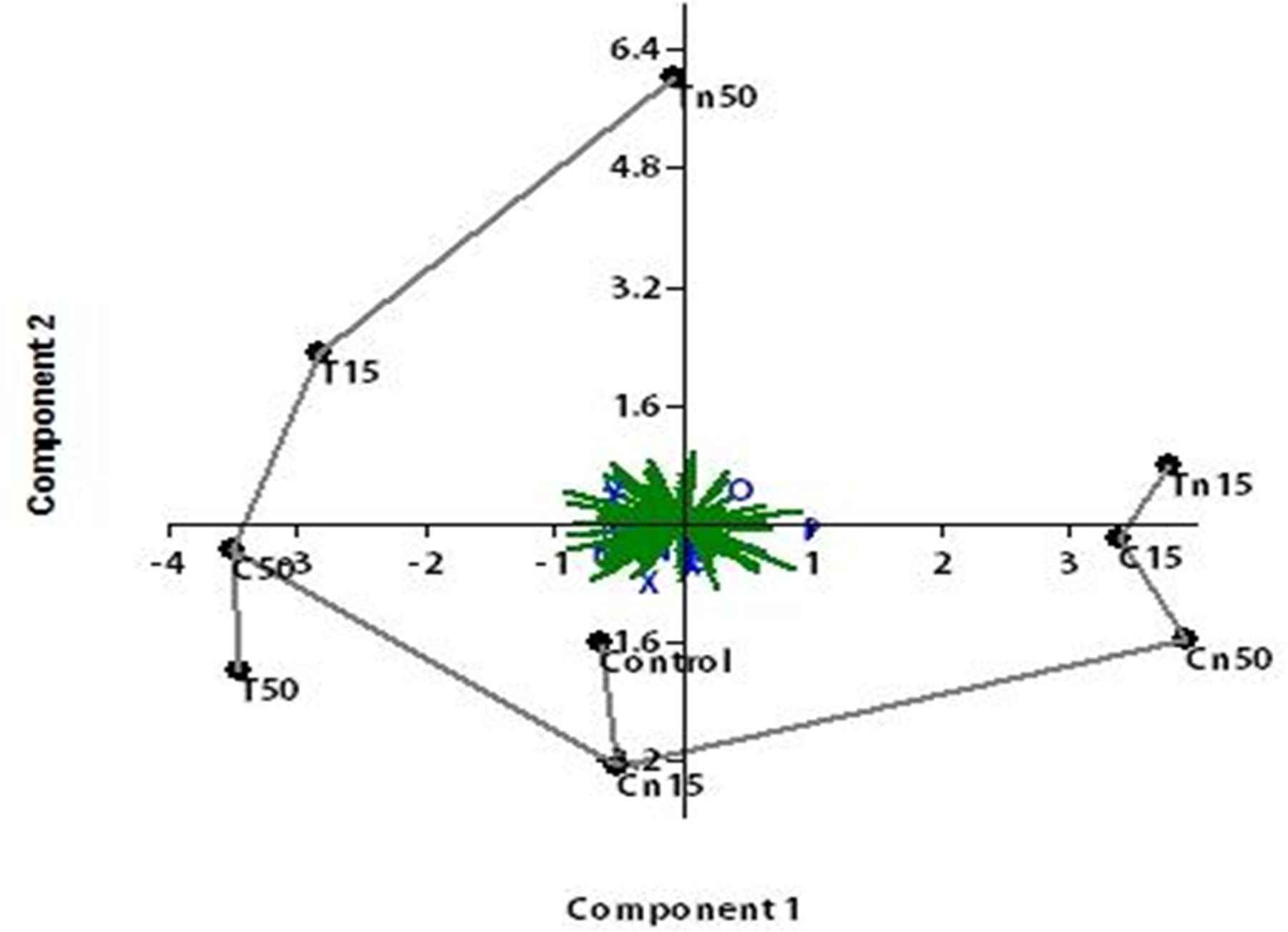
Figure 8. A representative agarose gel where PCR products of the 15 ISSR primers for the nine treatments. Image Credit: Awad, et al., 2022
Discussion
The ISSR-PCR approach was utilized as an efficient marker to evaluate the degrees of genetic mutagenicity in second instar larvae exposed to several insecticidal treatments (chlorantraniliprole, thiocyclam, and their nano-forms) to the untreated control. Compared to the control, the LC15 of nano-chlorantraniliprole treatment had the least mutagenic insecticidal effects on insect DNA.
The LC50 of nano-chlorantraniliprole had the highest mutagenic impact, followed by the LC50 of nano-thiocyclam. The PCA analysis of ISSR data yielded a similar outcome to the dendrogram topology. Furthermore, the mutagenic effects of nano-chlorantraniliprole LC15 and thiocyclam LC50 were shown to be nearly identical to the control.
The polymorphic variances in ISSR patterns are thought to be due to alterations in primer binding sites or DNA architecture, as well as DNA damage caused by pesticide treatment. Furthermore, it might be caused by DNA replication blockage, the existence of a significant number of chromosomal lesions, such as major rearrangements (e.g., deletion, inversion, or translocation), or unrepaired DNA damage caused by direct insecticide exposure.
The ISSR investigations proved their capacity to identify DNA damage and alterations induced by the pesticides tested.
Conclusion
To summarize, insecticide monomerization was created to increase the biological activity of traditional insecticides. The findings are a potential step forward in the development of safe and effective nano-insecticides. More research is needed to understand their environmental impact. This study is the first of its kind to look at the genome-wide DNA mutability, biochemical impacts, and toxicity levels of thiocyclam, chlorantraniliprole, and their nano-forms for A. ipsilon control.
Journal Summary
Awad, M., Ibrahim, E. D. S., Osman, E. I., Elmenofy, W. H., Mahmoud, A. W. M., Atia, M. A., Moustafa, M. A. (2022). Nano-insecticides against the black cutworm Agrotis ipsilon (Lepidoptera: Noctuidae): Toxicity, development, enzyme activity, and DNA mutagenicity. PloS one, 17(2), p. e0254285.
References and Further Reading
- Binning, R. R., et al. (2015) Susceptibility to Bt proteins is not required for Agrotis ipsilon aversion to Bt maize. Pest Management Science, 71, pp. 601–606. doi.org/10.1002/ps.3901.
- Abd El-Aziz, S. E., et al. (2007) Chemical composition of Ocimum americanum essential oil and its biological effects against, Agrotis ipsilon, (Lepidoptera: Noctuidae). Research Journal of Agriculture and Biological Sciences,3, pp. 740–747.
- Amin, A. H., et al., (2019) Youssef Efficiency of Nano-formulations of neem and peppermint oils on the bionomics and enzymatic activities of Agrotisipsilon larvae (Lepidoptera: Noctuidae). International Journal of Natural Resources and Technologies, 4, p. 102. doi.org/10.11648/j.ijnrem.20190405.11.
- Guedes, C &Siqueira, A (2012) The tomato borer Tutaabsoluta: insecticide resistance and control failure. Plant Science Review, 7, pp. 1–7. doi.org/10.1371/journal.pone.0254285.
- Kah, M (2015) Nanopesticides and nanofertilizers: emerging contaminants or opportunities for risk mitigation? Frontiers in Chemistry, 3, p. 64. doi.org/10.3389/fchem.2015.00064.
- Worrall, E. A., et al. (2018) Nanotechnology for plant disease management. Journal of Agronomy, 8, p. 285. doi.org/10.3390/agronomy8120285.
- Yan, S., et al. (2021) Nanometerization of thiamethoxam by a cationic star polymer nanocarrier efficiently enhances the contact and plant-uptake dependent stomach toxicity against green peach aphids. Pest Management Science,77, pp. 1954–1962. doi.org/10.1111/1744-7917.12953.
- Kah, M & Hofmann, T (2014) Nanopesticide research: current trends and future priorities. Environment International, 63, pp. 224–235. doi.org/10.1016/j.envint.2013.11.015.
- Pérez-de-Luque, A & Rubiales, D (2009) Nanotechnology for parasitic plant control. Pest Management Science, 65, pp. 540–545. doi.org/10.1002/ps.1732.
- Chaturvedi, M., et al. (2014) Tissue inhibitor of matrix metalloproteinases-1 loaded poly (lactic-co-glycolic acid) nanoparticles for delivery across the blood-brain barrier. International Journal of Nanomedicine, 9, pp. 575–588. doi.org/10.2147/IJN.S54750.
- Kandil, M. A., et al. (2020) Lethal and sublethal effects of bio-and chemical insecticides on the tomato leaf miner, Tuta absoluta (Meyrick) (Lepidoptera: Gelechiidae). Egyptian Journal of Biological Pest control, 30, pp. 1–7.doi.org/10.1186/s41938-020-00278-1.
- Kodrík, D., et al. (2015) Hormonal regulation of response to oxidative stress in insects. International Journal of Molecular Sciences, 16, pp. 25788–25816. doi.org/10.3390/ijms161025788.
- Martindale, J L & Holbrook, N J (2002) Cellular response to oxidative stress: signaling for suicide and survival. Journal of Cellular Physiology, 192, pp. 1–15. doi.org/10.1002/jcp.10119.
- Felton, G W & Summers, C B (1995) Antioxidant systems in insects. Archives of Insect Biochemistry and Physiology, 29, pp. 187–197. doi.org/10.1002/arch.940290208.
- Rudnev, II (1999) Antioxidant system of black sea animals in early development. Comparative Biochemistry & Physiology, 122, pp. 265–271. doi.org/10.1016/S0742-8413(98)10121-4.
- Weirich, G. F., et al. (2002) Antioxidant enzymes in the honey bee, Apismellifera Apidologie, 33, pp. 3–14. doi.org/10.1051/apido:2001001.
- Afiyanti, M & He, H-J (2014) Gatalase activity is modulated by calcium and calmodulin in detached mature leaves of sweet potato. Journal of Plant Physiology, 171, pp. 35–47. doi.org/10.1016/j.jplph.2013.10.003.
- Yoshida, K., et al. (2003) Molecular biology and application of plant peroxidase genes. Applied Microbiology and Biotechnology, 60, pp. 665–670. doi.org/10.1007/s00253-002-1157-7.
- Couto, N., et al. (2016) The role of glutathione reductase and related enzymes on cellular redox homoeostasis network. Free Radical Biology and Medicine, 95, pp. 27–42. doi.org/10.1016/j.freeradbiomed.2016.02.028.
- Board, P G & Menon, D (2013) Glutathione transferases, regulators of cellular metabolism and physiology. Biochimicaet BiophysicaActa - General Subjects, 1830, pp. 3267–3288. doi.org/10.1016/j.bbagen.2012.11.019.
- Parsons, B. J., et al. (1997) Contrasting genetic diversity relationships are revealed in rice (Oryzasativa L.) using different marker types. Molecular Breeding, 3, pp. 115–125. doi.org/10.1023/A:1009635721319.
- Van Droogenbroeck, B., et al. (2004) Phylogeneticanalysis of the highland papayas (Vasconcellea) and alliedgenera (Caricaceae) using PCR-RFLP. Theoretical and Applied Genetics, 108, pp.1473–1486. doi.org/10.1007/s00122-003-1575-7.
- Joshi, P & Dhawan, V (2007) Assessment of genetic fidelity of micropropagated Swertiachirayita plantlets by ISSR marker assay. Biologia Plantarum, 5, pp. 22–26. doi.org/10.1007/s10535-007-0005-0.
- Perez de Castro, A., et al. (2007) Identification of a CAPS marker tightly linked to the tomato leaf curl disease resistance gene Ty-1 in tomato. European Journal of Plant Pathology, 117, pp. 347–356. doi.org/10.1007/s10658-007-9103-2.
- Lindroth, E J (2011) Population genetics of the western bean cutworm (Striacosta albicosta Smith) across the United States. Annals of the Entomological Society of America, 105, pp. 685–692. doi.org/10.1603/AN11084.
- Sharma, K., et al. (2008) ISSR marker-assisted selection of male and female plants in a promising dioecious crop: jojoba (Simmondsiachinensis). Plant Biotechnology Reports, 2, pp. 239–243. doi.org/10.1007/s11816-008-0070-7.
- Hundsdoerfer, A K & Wink, M (2005) New source of genetic polymorphisms in Lepidoptera. Zeitschriftfür Naturforschung, 60, pp. 618–624. doi.org/10.1515/znc-2005-7-818.
- De Oliveira, J L., et al. (2015) Solid lipid nanoparticles co-loaded with simazine and atrazine: Preparation, characterization, and evaluation of herbicidal activity. Journal of Agricultural and Food Chemistry, 63, pp. 422–432. doi.org/10.1021/jf5059045.
- Cota-Arriola, O., et al. (2013) Controlled release matrices and micro/nanoparticles of chitosan with antimicrobial potential: Development of new strategies for microbial control in agriculture. Journal of the Science of Food and Agriculture, 93, pp. 1525–1536. doi.org/10.1002/jsfa.6060.
- Xu, Z. P., et al. (2006) Stable suspension of layered double hydroxide nanoparticles in aqueous solution. Journal of the American Chemical Society, 128, pp. 36–37. doi.org/10.1021/ja056652a.
- Moustafa, M. A. M., et al. (2021) Toxicity and sublethal effects of chlorantraniliprole and indoxacarb on Spodopteralittoralis (Lepidoptera: Noctuidae). Applied Entomology and Zoology, 56, pp. 115–124.doi.org/10.1007/s13355-020-00721-7.
- Hamada, H. M., et al. (2018) Insecticidal activity of garlic (Allium sativum) and ginger (Zingiberofficinale) oils on the cotton leafworm, Spodopteralittoralis (Boisd) (Lepidoptera: Noctuidae). African Entomology, 26, pp. 84–94. doi.org/10.1371/journal.pone.0254285.
- Moustafa, M. M. A., et al. (2016) Sublethal effects of spinosad and emamectin benzoate on larval development and reproductive activities of the cabbage moth, Mamestrabrassicae L. (Lepidoptera: Noctuidae). Crop Protection, 90, pp. 197–204. doi.org/10.1016/j.cropro.2016.09.004.
- Misra, H P & Fridovich, I (1972) The role of superoxide anion in the autoxidation of epinephrine and a simple assay for superoxide dismutase. Journal of Biological Chemistry, 247, pp. 3170–3175. doi.org/10.1016/S0021-9258(19)45228-9.
- Aebi, H (1984) Catalase in vitro. Methods in enzymology, 105, pp. 121–126. doi.org/10.1016/S0076-6879(84)05016-3.
- Ohkawa, H., et al. (1979) Assay for lipid peroxides in animal tissues by thiobarbituric acid reaction. Analytical Biochemistry,95, pp. 351–358. doi.org/10.1016/0003-2697(79)90738-3.
- Goldberg, D M & Spooner, R J (1983) Glutathione reductase. Methods in enzymology, 3, pp. 258–265. doi.org/10.1016/0003-2697(90)90668-Y.
- Atia, M. A., et al. (2017) Development of sex-specific PCR-based markers in date palm. Methods Molecular Biology, 1638, pp. 227–244. doi.org/10.1007/978-1-4939-7159-6_20.
- Atia, M. A., et al. (2017) Assessing date palm genetic diversity using different molecular markers. Methods in Molecular Biology, 1638, pp. 125–142. doi.org/10.1007/978-1-4939-7159-6_12.
- Rousseeuw, P J (1987) Silhouettes: a graphical aid to the interpretation and validation of cluster analysis. Journal of Computational and Applied Mathematics, 20, pp. 53–65. doi.org/10.1016/0377-0427(87)90125-7.
- Wickelmaier, F (2003) An introduction to MDS. Sound Quality Research Unit, Aalborg University, 46, pp. 1–26.
- Abouseadaa, H. H., et al. (2020) Gene-targeted molecular phylogeny, phytochemical profiling, and antioxidant activity of nine species belonging to the family Cactaceae. Saudi Journal of Biological Sciences, 27, pp. 1649–58. doi.org/10.1016/j.sjbs.2020.03.007.
- Jaccard, P (1908) Nouvelles recherché sur la distribution florale. Bulletin de la Sociétévaudoise des sciences naturelles, 44, pp. 223–70.
- Atia, M. A., et al. (2016) Genome-wide in silico analysis, characterization and identification of microsatellites in Spodopteralittoralis multiple nucleopolyhedrovirus (SpliMNPV). Scientific Reports, 6, pp. 1–9. doi.org/10.1038/srep33741.
- Liu, B H (2017) Statistical genomics: linkage, mapping, and QTL analysis. CRC press.
- Botstein, D., et al. (1980) Construction of a genetic linkage map in man using restriction fragment length polymorphisms. American Journal of Human Genetics, 32, p. 314.
- Powell, W., et al. (1996) The comparison of RFLP, RAPD, AFLP and SSR (microsatellite) markers for germplasm analysis. Molecular Breeding, 2, pp. 225–38. doi.org/10.1007/BF00564200.
- Tessier, C., et al. (1999) Optimization of the choice of molecular markers for varietal identification in Vitisvinifera L. Theoretical and Applied Genetics, 98, pp. 171–177. doi.org/10.1007/s001220051054.
- Prevost, A & Wilkinson, M J (1999) A new system of comparing PCR primers applied to ISSR fingerprinting of potato cultivars. Theoretical and Applied Genetics, 98, pp. 107–12. doi.org/10.1007/s001220051046.
- Gopal, M., et al. (2012) Nano-pesticides—A recent approach for pest control. Journal of Plant Protection Sciences, 4, pp. 1–7.
- Gopal, M., et al. (2012) Nano-pesticides—A recent approach for pest control. The Journal of Plant Protection Sciences, 4, pp.1–7.
- Insecticide Resistance Action Committee. IRAC Mode of Action Classification Scheme. IRAC. 2020; v 9.4.
- Cordova, D., et al. (2006) A new class of insecticides with a novel mode of action, ryanodine receptor activation. Pesticide Biochemistry and Physiology, 84, pp. 196–214A.
- Lihl, C., et al. (2020) Compound-specific chlorine isotope fractionation in biodegradation of atrazine. Environmental Science: Processes & Impacts, 3, pp. 792–801. doi.org/10.1039/C9EM00503J.
- Camargo, J A (1991). Toxic effects of residual chlorine on larvae of Hydropsyche pellucidula (Trichoptera, Hydropsychidae): A proposal of biological indicator. Bulletin of Environmental Contamination and Toxicology, 47, pp. 261–265. doi.org/10.1007/BF01688649.
- Institute of Medicine of the National Academies (2005) Chapter 7: Dietary Reference Intakes for Water, Potassium, Sodium, Chloride, and Sulfate; National Academies Press: Washington, DC.
- UConn Home and Garden Education Center (2017).
- Radwan, E M &Taha, H S (2017). Efficacy of certain pesticides against larvae of Tomato Leafminer, Tuta absoluta (Meyrick) (Lepidoptera: Gelechiidae). Egyptian Academic Journal of Biological Sciences, A9, pp. 81–95. doi.org/10.21608/eajbsc.2017.13675.
- Hosseinzadeh, A., et al. (2019) Efficacy of bio-insecticides on Tuta absoluta (Meyrick) (Lep.: Gelechiidae) in laboratory and field conditions. Agricultural Engineering International, 21, pp. 164–170.
- Lai, T & Su, J Y (2011) Effects of chlorantraniliprole on development and reproduction of beet armyworm, Spodoptera exigua (Hübner). Journal of Pest Science, 84, pp. 381–386. doi.org/10.1007/s10340-011-0366-1.
- Cao, G. C., et al. (2010) Toxicity of chlorantraniliprole to Cry1Ac-susceptible and resistant strains of Helicoverpa armigera. Pesticide Biochemistry and Physiology, 98, pp. 99–103. doi.org/10.1016/j.pestbp.2010.05.006.
- He, F., et al. (2019) Chlorantraniliprole against the black cutworm Agrotis ipsilon (Lepidoptera: Noctuidae): from biochemical/ physiological to demographic responses. Scientific Reports, 9, pp. 1–17. doi.org/10.1038/s41598-019-46915-0.
- Yin, X.-H., et al. (2008) Sublethal effects of spinosad on Plutella xylostella (Lepidoptera: Yponomeutidae). Crop Protection, 27, pp.1385–1391. doi.org/10.1016/j.cropro.2008.05.008.
- Wang, P., et al. (2017) Sublethal effects of thiamethoxam on the demographic parameters of Myzus persicae (Hemiptera: Aphididae). Journal of Economic Entomology, 110, pp. 1750–1754. doi.org/10.1093/jee/tox112.
- Lutz, A. L., et al. (2018) Lethal and sublethal effects of chlorantraniliprole on Spodoptera cosmioides (Lepidoptera: Noctuidae). Pest Management Science, 74, pp. 2817–2821. doi.org/10.1002/ps.5070.
- El-Dewy, M E H (2017) Influence of some novel insecticides on physiological and biological aspects of Spodoptera littoralis (Boisduval). Alexandria Science Exchange Journal, 38, pp. 250–258. doi.org/10.21608/asejaiqjsae.2017.3353.
- Han, W. S., et al. (2012) Residual toxicity and sublethal effects of chlorantraniliprole on Plutella xylostella (Lepidoptera: Plutellidae). Pest Management Science, 68, pp. 1184–1190. doi.org/10.1002/ps.3282.
- Banerjee, B. D., et al. (2001) Pesticide-induced oxidative stress: perspectives and trends. Reviews on Environmental Health, 16, pp. 1–36. doi.org/10.1515/reveh.2001.16.1.1.
- Afolabi, O. K., et al. (2019) Oxidative stress and inflammation following sub-lethal oral exposure of cypermethrin in rats: Mitigating potential of epicatechin. Heliyon, 5, pp. 125–134. doi.org/10.1016/j.heliyon.2019.e02274.
- Bednářová, A., et al. (2013) Adipokinetic hormone exerts its anti-oxidative effects using a conserved signal-transduction mechanism involving both PKC and cAMP by mobilizing extra- and intracellular Ca2+ stores. Comparative Biochemistry and Physiology, 158, pp. 142–149. doi.org/10.1016/j.cbpc.2013.07.002.
- Valavanidis, T., et al. (2006) Scoullos Molecular biomarkers of oxidative stress in aquatic organisms in relation to toxic environmental pollutants. Ecotoxicology and Environmental Safety, 64, pp. 178–189. doi.org/10.1016/j.ecoenv.2005.03.013.
- Draper, H. H., et al. (1993) Comparative evaluation of thiobarbituric acid methods for the determination of malondialdehydein biological materials. Free Radical Biology and Medicine, 15, pp. 353–363.
- Wu, G. C., et al. (2017) Individual polyphenolic profiles and antioxidant activity in sorghum grains are influenced by very low and high solar UV radiation and genotype. Journal of Cereal Science, 77, pp. 17–23. doi.org/10.1016/j.jcs.2017.07.014.
- Zhang, Q. M., et al. (2013) Oxidative stress and lipid peroxidation in the earthworm Eiseniafetida induced by low doses of fomesafen. Environmental Science and Pollution Research, 20, pp. 201–208. doi.org/10.1007/s11356-012-0962-5.
- Loewe, L & Hill, WG (2010) The population genetics of mutations: good, bad and in different. Philosophical Transactions of the Royal Society B, 365, pp. 1153–1167. doi.org/10.1098%2Frstb.2009.0317.
- Michod, R E (1995) Eros and Evolution. A Natural Philosophy of Sex, Addison-Wesley Publishing, USA.