Bio-AFM is a cutting-edge technique rooted in the rich history of atomic force microscopy. Here, we discuss its applications to nanoscience.
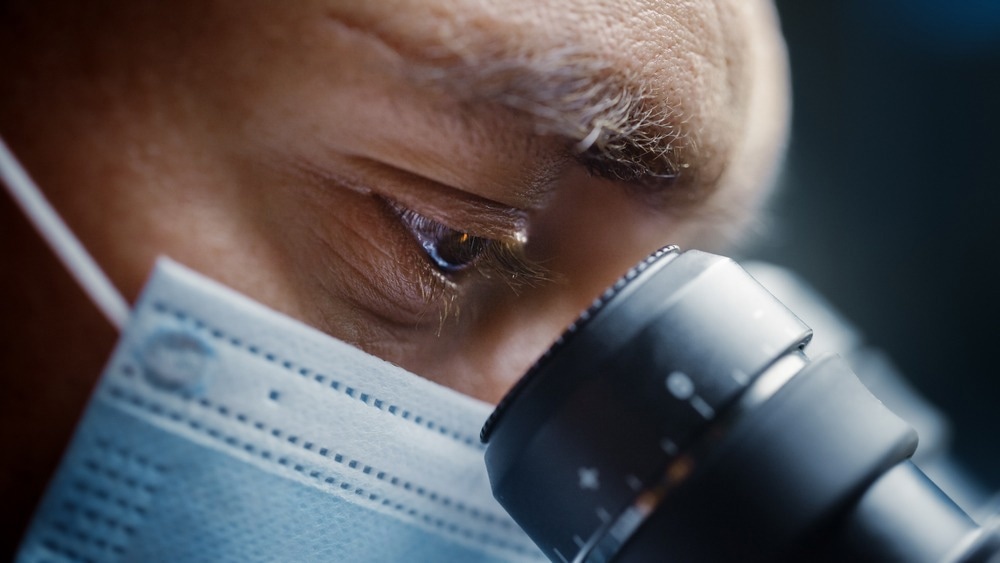
Image Credit: Gorodenkoff/Shutterstock.com
Studying nanoscale biological specimens has been important for growing our understanding of humans and the world around us. Today, we rely on advancements in our knowledge of biological systems to help us prevent, diagnose, and treat disease.
Bio-AFM is one such technique that is helping to move this field of science forward. In particular, it is becoming increasingly used in nanoscience applications, which will likely be important to developing more effective gene therapy vehicles.
A Brief History of Bio-AFM
The history of the biological atomic force microscope (bio-AFM) dates back to the early 1980s with the development of the scanning tunneling microscope (STM) by IBM scientists Gerd Binning and Heinrich Roher. Their invention, which visualized the atomic structure of a sample surface, earned them the Nobel Prize in Physics in 1986.
Following the success of the STM, the atomic force microscope (AFM) was developed to broaden the range of samples that could be investigated, given that the STM can only be applied to conductive or semi-conductive samples. In 1985, the AFM was invented by IBM scientists, and in 1998, the first commercial AFMs were created by researchers at Stanford.
Bio-AFM is the process of applying AFM to biological samples. Over the years, AFM has been used to study genetic material such as DNA and chromosomes, bacteria, viruses, mammalian cells, proteins, peptides, and biological membranes.
How Does Bio-AFM Work?
The underlying mechanism of AFM technology is based on detecting forces that act between the sharp probe and the sample’s surface. To detect these forces, the probe is attached to a flexible cantilever, and the motion of the cantilever’s movements is measured.
While there are several methods to obtain this measurement, the most common in modern science is to use lasers. Laser light is reflected from the cantilever onto position-sensitive detectors.
By measuring the deflection of the cantilever as the tip is scanned across the surface, information on the ‘stiffness’ of the sample can be obtained. These stiffness measurements, which can be translated into measurements of interactive forces, can be used to look at how different kinds of biological species interact, as well as provide a way of imaging the biological specimen.
What makes bio-AFM an excellent tool for looking at nanoscale biological samples is its very high spatial resolution. Most viruses are nanoscale in size and standard optical microscopy techniques are not sufficiently high resolution to see the virus and cannot recover information on different types of interaction. Bio-AFM also allows for studying biological samples in their native, liquid environments, often with relatively minimal sample preparation.
Applications of Bio-AFM in Nanoscience
Over the past decade, AFM has been increasingly used to obtain nanostructural details of biological samples. The high spatial resolution means that even very small structural details in viruses, proteins and cells can be resolved. While there are challenges associated with measuring directly inside cells, cell biology routinely makes use of bio-AFM techniques as a way of testing the mechanical properties of cells.
The types of mechanical properties measured include cell stiffness and viscoelasticity, and changes in the mechanical properties of the cell membrane. Nanoscale bio-AFM has also been used to reveal DNA structures, explore DNA condensation mechanisms and various gene-packaging materials, and investigate interactions between molecules at the molecular level.
The high spatial resolution and compatibility with several kinds of sample environments mean bio-AFM can be used to look at how single-strand or more standard DNA behaves at regions such as interfaces for developing new biomarkers and tags.
One example includes the use of bio-AFM to detect carboxylated nanodiamond on human cells as a way of screening for cytotoxicity of particular carbon nanotube objects. With the rapid development of new nanoscale carbon materials, there has been concern about the health risks that these new compounds could show. Bio-AFM has made it possible to visualize whether or not certain shaped nanotubes can penetrate cells.
Organ-on-a-chip nanoscale machines are another technological avenue that is being enabled by bio-AFM. Nanoscale machines are used to try and control the spatial and temporal movements of nanoscale objects like cells and tissues and bio-AFM can be used to characterize the material properties for what structures may have the right mechanical properties to be used for this application.
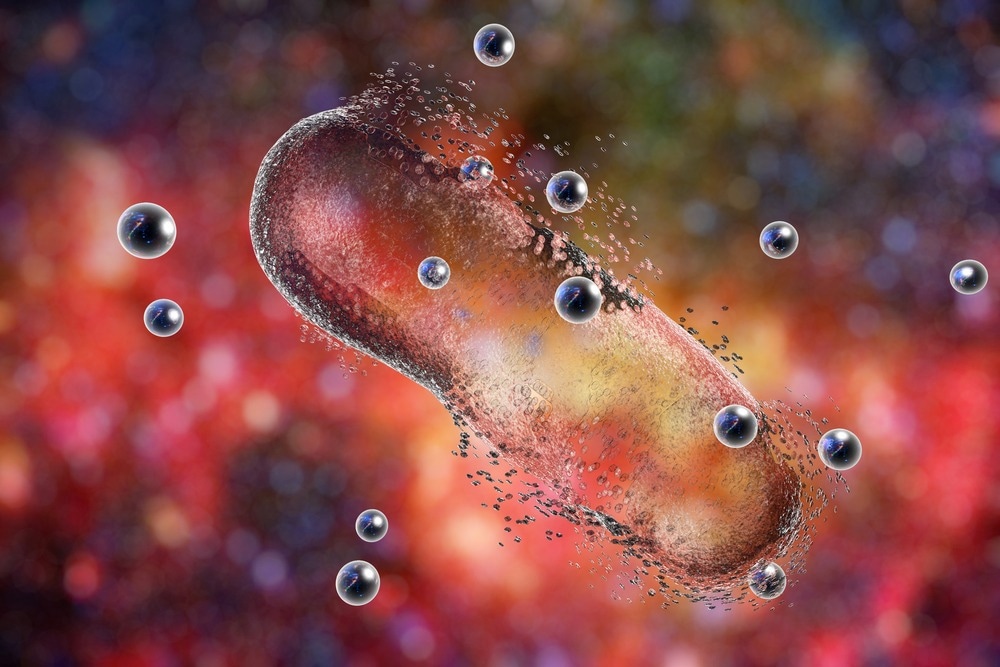
Image Credit: Kateryna Kon/Shutterstock.com
The ability to investigate surfaces and monitor cell and pathogen interactions has made bio-AFM a very valuable tool in therapeutic development. One of the biggest challenges in drug design can be finding ways to deliver the therapeutic to the biological target. Bio-AFM has been used to look at how silver nanoparticles can help destroy pathogens and how other types of bacteria can be used to manufacture the right shape and size nanoparticles with which to do this. Bio-AFM is one potential path for such sizing processes and checking the purity of complex synthetic pathways.
Most disease diagnosis is carried out by looking at morphological changes to tissues, but disease and normal cell function often result in changes in the mechanical properties of the cell, not just its appearance. Many viruses themselves also undergo changes to their mechanical properties during the infection process as well. The additional information provided by nanoscale bio-AFM may open a new range of diagnostic tools and further our understanding of the mechanics underlying biological processes.
How Does Bio-AFM Compare to Other AFM Techniques?
The use of AFM in biological contexts leverages working features of AFM that are particularly suited to investigating biological samples. For example, bio-AFM often operates in liquid environments rather than in a vacuum or air to ensure that the appropriate conditions for biological samples can be maintained.
However, mostly, bio-AFM and AFM rely on similar methodologies and techniques; the difference lies in the sample and how the results can be interpreted.
In the coming years, we can potentially expect developments in the technology to make it more specialized for studying biological samples. Some manufacturers have already begun producing equipment that is specific to bio-AFM applications.
In the future, we will likely see further technological developments as the demands of the industry evolve.
References and Further Reading
Allison, D.P. et al. (2010) “Atomic Force Microscopy of biological samples,” WIREs Nanomedicine and Nanobiotechnology, 2(6), pp. 618–634. https://doi.org/10.1002/wnan.104.
Bio AFM [Online]. Nanonics. Available at: https://www.nanonics.co.il/wiki/bio-afm
Chang, K.-C. et al. (2012) “Atomic Force Microscopy in biology and Biomedicine,” Tzu Chi Medical Journal, 24(4), pp. 162–169. https://doi.org/10.1016/j.tcmj.2012.08.002.
Cypher ES: Atomic Force Microscopy with Environmental Control, Uniquely Well Implemented [Online]. Oxford Instruments. Available at: https://afm.oxinst.com/outreach/environmental-control-with-cypher-es-afm
Yang, Ruiguo et al. (2011) “The Emergence of AFM Applications to Cell Biology: How new technologies are facilitating investigation of human cells in health and disease at the nanoscale.” Journal of nanoscience letters, 1(2), pp. 87-101. https://www.ncbi.nlm.nih.gov/pmc/articles/PMC3886279/
Disclaimer: The views expressed here are those of the author expressed in their private capacity and do not necessarily represent the views of AZoM.com Limited T/A AZoNetwork the owner and operator of this website. This disclaimer forms part of the Terms and conditions of use of this website.