In this article, we delve into the complexities of antibiotic-based environmental pollution and explore how nanoparticles offer a promising solution for effective water purification while also examining the advantages and challenges associated with their use.
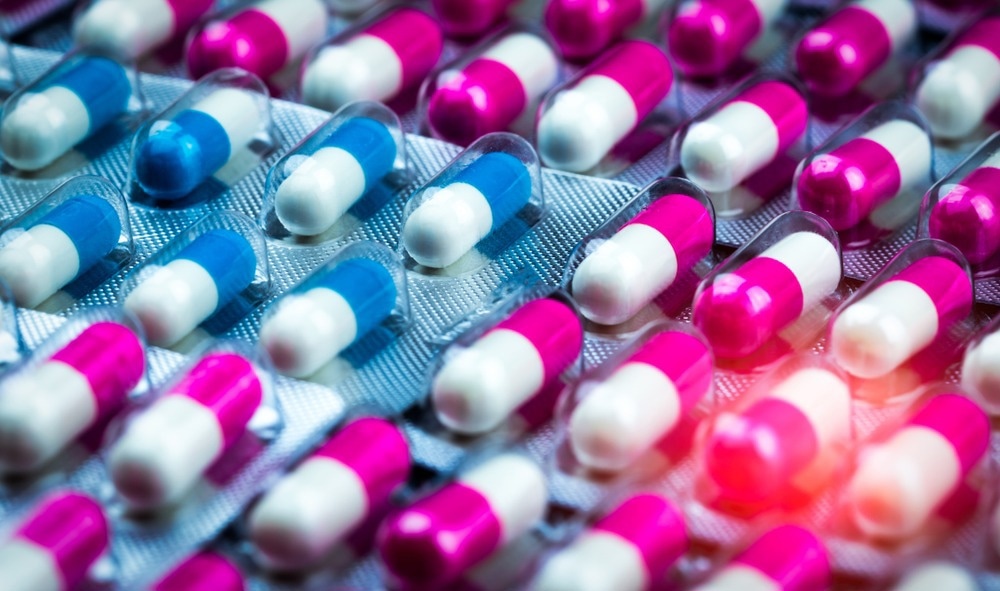
Image Credit: Fahroni/Shutterstock.com
Why are Antibiotics Classed as Environmental Pollutants?
Active pharmaceutical compounds are considered emerging pollutants within the water supply, particularly in locations with high population density and already limited water supply, necessitating higher rates of water renewal.
Activate pharmaceutical compounds such as antibiotics that enter the water system and surrounding environment largely originate from human excretion, as compounds filtered by the renal and hepatobiliary system are eventually removed via the urine and feces. In particular, antibiotic environmental pollution raises concerns about growing antibiotic resistance, as continuous low-level exposure to antibiotics strongly promotes adaptation in bacteria.
Nanoparticles constructed from a variety of materials are promising antibiotic agents that generally do not promote resistance, as their mechanism of action usually involves catastrophic destruction of the bacterial cell membrane and biofilm disruption by the strong surface energies of the particle, against which adaptation has not been observed.
The high surface energy of metallic and metal oxide nanoparticles is a result of delocalized electrons that are free to engage in various exchanges with local molecules, generating free radicals that may go on to degrade organic compounds.
What are the Advantages of Nanoparticle-Based Water Purification?
Typical physical, chemical, and biological means of removing trace quantities of active pharmaceuticals from wastewater are expensive to establish and maintain, frequently have large power requirements, and generally incompletely remove trace contaminants at the required scale.
Compared to bulk components constructed of the same materials, nanoparticles possess much greater surface area, and are more capable of freely mixing with wastewater while being easily removed by filtration, centrifugation, or magnetism. Nanoscale objects also often demonstrate differing physicochemical properties to their bulk counterparts, in the case of metallic particles constructed from copper or silver significantly enhancing surface energies as a result of delocalized and expanded d-orbitals.
Nanoparticles are also of the appropriate scale to generate severe surface curvature within the bacterial cell wall or membrane when in close proximity or during ingress. They can also be meaningfully functionalized with molecules that assist in bacterial interaction or antibiotic efficacy. The high energy surface can also act as a catalyst in various electron exchange interactions, namely free radical generation and the degradation of organic molecules.
On the other hand, nanoparticles themselves often exhibit toxicity towards the environment or humans, particularly when they also demonstrate strong antibiotic or catalytic efficacy, and thus must be carefully utilized during water treatment. Additionally, nanoparticle manufacture often requires high temperatures and toxic reagents, posing cost and environmental concerns. The green synthesis of metallic and metal oxide nanoparticles can be achieved using extracts from plants at slightly elevated or room temperature, which typically act as a reducing agent to facilitate the bottom-up synthesis of particles from metal salts. Nvilu et al. (2023) used this method, specifically, the plant Parthenium hysterophorus, an invasive weed that grows in many locations around the world, was extracted into an aqueous solution and mixed with copper (II) sulfate to produce roughly spherical CuO nanoparticles with polydisperse diameters of around 30 – 100 nm.
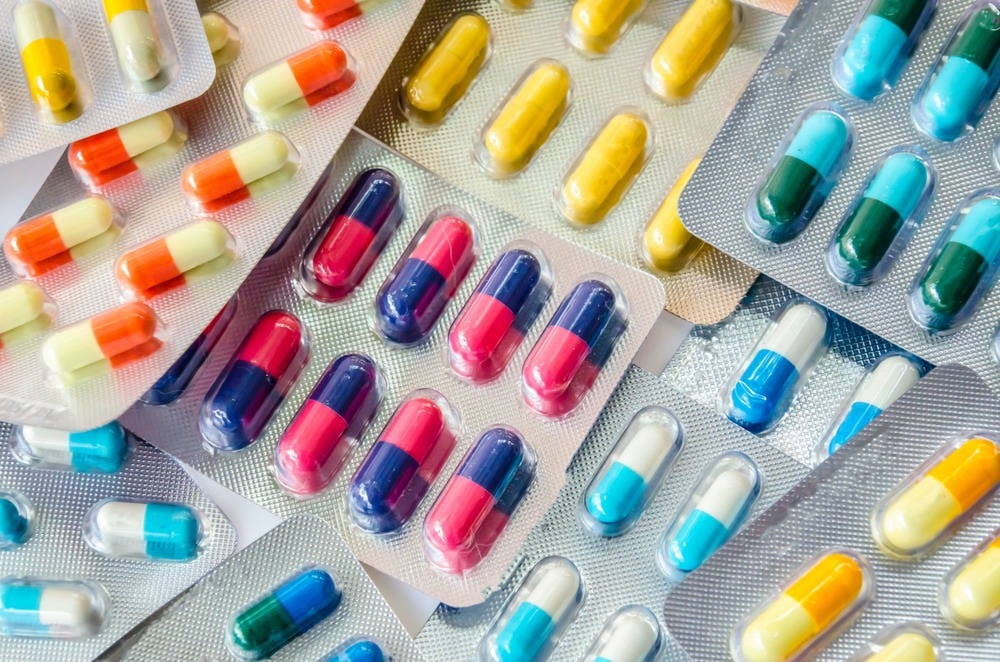
Image Credit: nokwalai/Shutterstock.com
How do Nanoparticles Degrade Waste Active Pharmaceutical Compounds?
Rifampicin is an antibiotic used to treat several types of bacterial infection, perhaps most prominently tuberculosis. Around 30% of ingested rifampicin is excreted in the urine, and a further ~60% in the feces, and thus is a major source of active pharmaceutical compound wastewater contamination, particularly in tuberculosis-affected regions, which are typically less developed and thus already lack rigorous wastewater treatment facilities.
The abovementioned study by Nvilu et al. (2023) tested the antibiotic degradation efficacy of the produced CuO nanoparticles towards rifampicin, finding that concentrations of 10 – 50 mg/L CuO were capable of degrading 93-99% rifampicin in solution, efficacy increasing with concentration.
The high surface area of CuO nanoparticles provides a large number of adsorption sites onto which rifampicin can bind, while the nanoparticle also acts as a catalyst in the generation of reactive oxygen species and other free radicals; from rifampicin itself, surrounding water molecules, dissolved oxygen, and other minor sources.
Numerous types of condensation or hydrolysis reaction may be initiated by free radicals in an aqueous environment, causing the breaking up, cyclization, or otherwise inactivation of rifampicin or other active pharmaceutical compounds. Interestingly, pH was also found to play a role in rifampicin degradation by CuO nanoparticles, the specific pH of 5 causing a decline in efficacy from ~98% to only 94%, keeping other conditions the same. This is due to the change in H+/OH- ion population as pH shifts, and the subsequent change in the rate of reactive species generation. Similarly, warmer temperatures promote rifampicin degradation as nanoparticle-drug interactions occur more frequently and the subsequent rate of reaction causing degradation is increased, related to increased Brownian motion.
In detail, the process of active pharmaceutical compound degradation by metallic or metal oxide nanoparticles proceeds firstly by excitation of the nanoparticle by incident light, which causes the promotion of an electron from the valence to the conduction band, generating an electron hole in the former.
By electron exchange, the conduction band electron can reduce molecular oxygen to oxygen radicals. At the same time, a water molecule is oxidized to produce hydroxyl radicals upon transferring an electron to the valence band electron-hole. Numerous points of the active pharmaceutical compound are then sensitive to attack by these excited species. However, the degree of efficacy will depend strongly on the molecular structure of the contaminant, as well as properties above such as relative concentration, temperature, and particle shape and size.
Reference and Further Reading
Nzilu, D. M., et al. (2023). Green synthesis of copper oxide nanoparticles and its efficiency in degradation of rifampicin antibiotic. Scientific Reports, 13(1). doi.org/10.1038/s41598-023-41119-z
Liu, L.-K., et al. (2016). The Structure of the Antibiotic Deactivating, N-hydroxylating Rifampicin Monooxygenase. Journal of Biological Chemistry, 291(41), pp. 21553–21562. doi.org/10.1074/jbc.m116.745315
Ozdal, M., & Gurkok, S. (2022). A Recent advances in nanoparticles as antibacterial agent. ADMET and DMPK. doi.org/10.5599/admet.1172
Disclaimer: The views expressed here are those of the author expressed in their private capacity and do not necessarily represent the views of AZoM.com Limited T/A AZoNetwork the owner and operator of this website. This disclaimer forms part of the Terms and conditions of use of this website.