Since its discovery in 2004, graphene has become a highly prized material in electronics applications owing to its unusual electronic structure.1 Although graphene is only composed of carbon atoms, what makes graphene’s material properties so different from other carbon allotropes, such as graphite, is the fact that it is a two-dimensional (2D) material.2
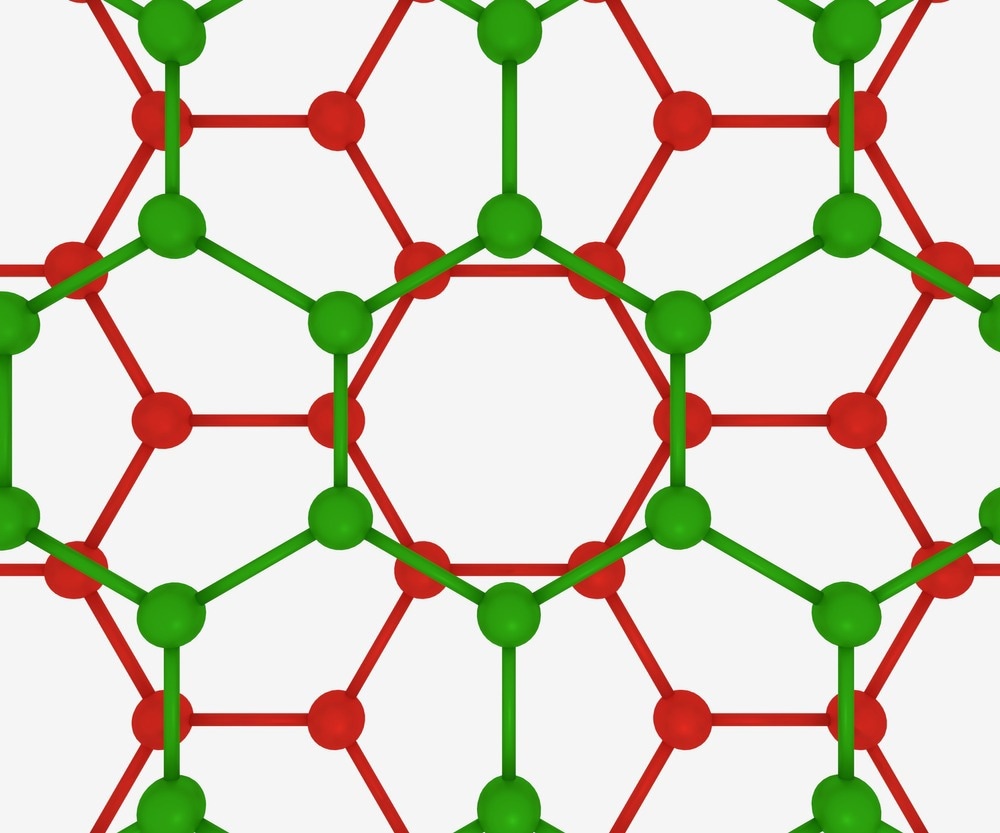
Image Credit: MZinchenko/Shutterstock.com
2D materials are atomically thin in one dimension with a periodically repeating unit cell in the other. Within a graphene sheet, the carbon atoms are bonded such that each carbon has essentially two free electrons that can be delocalized throughout the sheet-like structure and can act as a conductor.3 Overall, the thinness of the sheets results in a highly flexible materials, that have both excellent thermal and electrical conductivity, making them an excellent candidate for transistors.4
Many variants of monolayer graphene exist, with the introduction of dopants into the structure. Another way of tuning and modifying the properties of graphene is to make a bilayer material, where two coupled single layers of graphene are placed on top of each other. Bilayer graphene has the unique property that tits band gap can be tuned by using an external electric field, making a material where it is possible to control its properties not just through its synthesis but through its environmental conditions.5
Raman Spectroscopy
When developing new materials or characterizing and investigating their properties, it is important to have analytical techniques that are well-suited to the material type and can recover the required information.
Raman spectroscopy is a popular tool in materials science for quantitative and qualitative analysis of samples. In a Raman measurement, light interacts with the sample and the Raman scattered radiation is detected.
The Raman shift, or the change in the frequency of the detected radiation, can be used to work out the frequencies of the vibrational modes or electronic transitions in material. As the specific frequencies of vibrational modes in a chemical bond are dependent on the atoms involved and their local chemical environment.
Raman spectroscopy has been used to study both monolayer and bilayer graphene extensively.6 There are unique Raman ‘fingerprints’ for single, bilayer or few-layer graphene materials that can be used for identification of the sample type and, in the multilayer graphene materials, these fingerprints are sensitive to the changing interactions between the graphene layers.
Twist Angles
Combining 2D materials in layers and manipulating the relative orientation of the materials to create van der Waals heterostructures has been of great interest in the nanomaterials community. Often, the van der Waals heterostructures show distinctly different electrical, optical and mechanical properties from their constituent materials that are often dependent on the ‘twist angle’ between the two layers.7
As the Raman spectrum is sensitive to the electronic structure of graphene, research groups have been able to use Raman spectroscopy to study bilayer graphene materials with a variety of twist angles.7 The team observed that at very low twist angles, close to 0°, most sheets were in what is known as a Bernal-stacked form, where half of the atoms in the upper sheet lie over the center of the hexagon on the lower sheet.
As the twist angle begins to increase, the stacking arrangement begins to change until a ‘magic angle’ is reached – this angle is the point at which graphene is thought to display superconductive behavior. The Raman signals at this point showed a distinctive change in the lineshape that could be used to map the band structure of the system.
Twist Disorder
Other research groups have been using Raman spectroscopy to investigate the degree of disorder that occurs in the material around this magic angle.8 Bilayer graphene and the changes in the behavior of the band structure in this critical region are proving very interesting both in terms of applications that require development of better methods for controlling the twist angle, and in terms of fundamental questions about how many-particle systems behave. To further explore these questions, many research groups are complementing the Raman spectroscopy measurements with methods such as electron microscopy and modelling.8
As well as detailed investigations of the so-called G-band at this magic angle, other groups have been focusing on using Raman spectroscopy, often alongside infrared measurements, to investigate the structures and arrangements of particular sheet stacking configurations.9
The AA-stacking form of bilayer graphene is somewhat similar to the types of structure seen in graphite and is distinguishable using Raman spectroscopy.9 The team found that the band structures of the AA-stacked form were very different from other geometric arrangements of the layers, showing the importance of the inter-sheet interactions in determining the material's electronic properties.
Overall, Raman spectroscopy has proved a very important tool in characterizing the electronic and vibrational structure of bilayer graphene and other van der Waals heterostructures. Particularly, Raman spectroscopy is useful for understanding the relative orientations of the sheets and the interactions between them. Exploiting these properties will be a key part of practical applications for such materials.
References and Further Reading
Le Ferrand, H., et al. (2020). 3D Assembly of Graphene Nanomaterials for Advanced Electronics. Advanced Intelligent Systems, 2(5), p. 1900151. doi.org/10.1002/aisy.201900151
Novoselov, K. S., et al. (2004). Electric field effect in atomically thin carbon films. Science, 306(5696), pp. 666-669. doi.org/10.1126/science.1102896
Malik, R., et al.(2019). Chapter 16 - Hybridized Graphene for Chemical Sensing. Functionalized Graphene Nanocomposites and Their Derivatives. Elsevier Inc. doi.org/10.1016/B978-0-12-814548-7.00016-7
Yan, Z., et al. (2015). Thermal properties of graphene and few‐layer graphene applications in electronics. IET Circuits, Devices and Systems, 9(1), pp. 4–12. doi.org/10.1049/iet-cds.2014.0093
Castro, E. V., et al. (2008). Bilayer graphene: Gap tunability and edge properties. Journal of Physics: Conference Series, 129. doi.org/10.1088/1742-6596/129/1/012002
Ferrari, A. C., et al. (2006). Raman spectrum of graphene and graphene layers. Physical review letters, 97(18), p. 187401. doi.org/10.1103/PhysRevLett.97.187401
Barbosa, T. C., et al. (2022). Raman spectra of twisted bilayer graphene close to the magic angle. 2D Materials, 9, p. 025007. doi.org/10.1088/2053-1583/ac4af9
Andrei, E. Y., et al. (2020). Graphene bilayers with a twist. Nature Materials, 19(12), pp. 1265–1275. doi.org/10.1038/s41563-020-00840-0
Xu, Y., et al. (2010). Infrared and Raman spectra of AA-stacking bilayer graphene. Nanotechnology, 21(6), p. 065711. doi.org/10.1088/0957-4484/21/6/065711
Disclaimer: The views expressed here are those of the author expressed in their private capacity and do not necessarily represent the views of AZoM.com Limited T/A AZoNetwork the owner and operator of this website. This disclaimer forms part of the Terms and conditions of use of this website.