Utilizing a functional polymer coupled with disulfide-containing anchoring groups, a one-pot technique for manufacturing polymer-coated silver nanoparticles was established by the researchers in the latest article published in the journal ACS Omega.
Study: Highly Stable Polymer Coating on Silver Nanoparticles for Efficient Plasmonic Enhancement of Fluorescence. Image Credit: Terax Kriengsak/Shutterstock.com
Importance of Siler Nanoparticles
Silver nanoparticles are gaining popularity due to their wide variety of biomedical uses. Silver nanoparticles' antimicrobial activities have been utilized to inhibit bacterial development, involving surgical treatments, healing and burn care, and healthcare equipment. They are often utilized as sterilizing nanomaterials in consumer and medical items like fabrics, canned food bags, refrigerators panels, and hygiene products.
These nanomaterials have been shown to exhibit oxidative enzymatic capabilities for both biological agents like dyes and industrial chemicals like benzene. Silver nanoparticles are also commonly utilized as sensors for surface-enhanced Raman scattering (SERS) and metal-enhanced fluorescence (MEF).
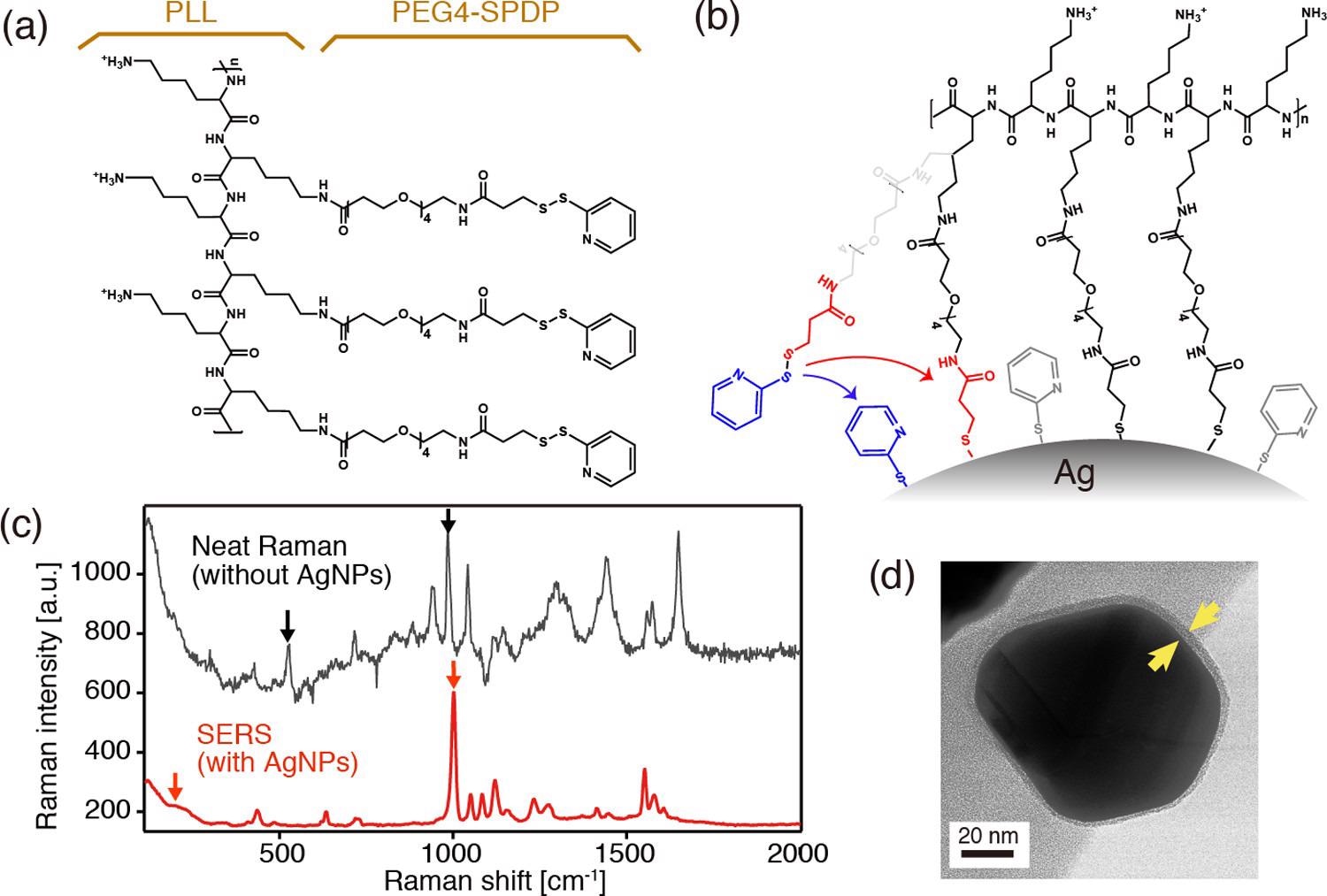
(a) Chemical structure of PLL in conjugation with PEG4-SPDP. (b) Schematics depicting the covalent binding of the PLL-PEG4-SPDP polymer through cleavage of disulfides. (c) Neat Raman and surface-enhanced Raman spectra of PLL-PEG4-SPDP measured with and without AgNPs, respectively. (d) TEM image of a PLL-coated core–shell nanoparticle. © Kato, R. et al., (2022)
Introduction to Plasmon-Enhanced Fluorescence
The study of plasmon enclosure of light to regions far smaller than wavelengths has cleared the way for strong augmentation strategies in visual spectroscopic methods. Fluorophore labels are combined with the tightly constrained plasmon resonance field in PEF.
Plasmon-enhanced fluorescence has piqued considerable interest in disease diagnosis because of its capacity for accurate biosensing.
In nanoparticles, localized surface plasmon resonances (LSPRs) generate regionally increased electrical fields that activate cellular fluorescence close to the nanoparticle. As the nanoparticle fluorophore separation distance reduces, the increased fluorescence grows considerably, allowing the detection of single-molecule fluorescence. When a fluorophore is in close contact with the interface of the nanostructured materials, spontaneous non-emissive transfer of energy from the fluorophore to the metal happens, resulting in fluorescence quenching.
Development of Antiquenching Spacers
So far, much work has been expended on the development of anti-quenching separators.
One of the most viable alternatives is the employment of core-shell nanostructures, which have metallic (plasmonic) centers and sufficiently thin nonmetallic exteriors. Because of its simple reaction sequence and excellent layer adaptability, silica is most typically used as a shell material.
Many groups have proven a basic chemical fabrication of silica films on any metallic nanoparticles, which has numerous uses in catalysts, ophthalmology, and semiconductors. The width and composition of outer sheets of silica core-shell nanoparticles may also be adjusted. Other insulating shell compounds, such as Al2O3 and ZnO, have been shown to impact quenching effectiveness based on their indices of refraction, in addition to silica.
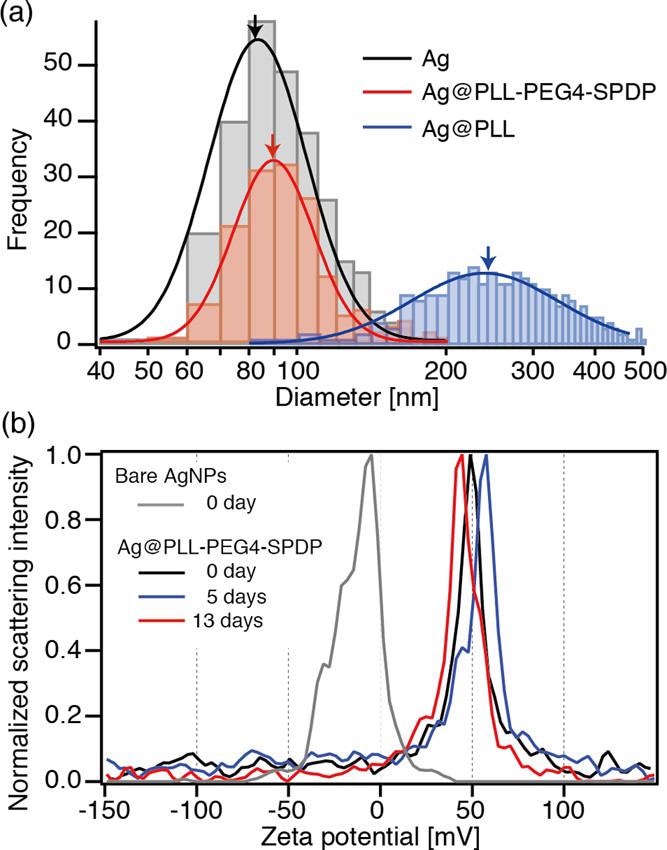
(a) Diameter distribution of bare Ag, Ag@PLL-PEG4-SPDP, and Ag@PLL nanoparticles, obtained by optical interferometric measurements. (b) Time-dependent ζ-potential measurements of bare Ag and Ag@PLL-PEG4-SPDP nanoparticles. © Kato, R. et al., (2022)
Effectiveness of Polymer Coatings
Even while silica-coated nanoparticles with a diversity of shell diameters are currently accessible, it is still difficult to manage the thickness below 5 nm in a reproducible manner. Furthermore, polymer shells have practical benefits in terms of biochemical durability, high compliance with a polymer matrix, and interface alteration adaptability for the coupling of a wide range of compounds, including macromolecules and pigments.
Polymer shells are often created using binding compounds and many processes, which necessitates the careful selection of crosslinking agents and optimization of variables. Furthermore, the polymer coating's durability should be increased to expand the usage of polymer-coated plasmonic particles for a spectrum of uses.
The researchers demonstrated a one-pot approach for encapsulating Ag nanoparticles with a multifunctional polymer containing positively charged hydrophilic amino groups.
Research Findings
The disulfide bonds in SPDP were expected to be severed because the PLL-PEG4-SPDP polymers were in close contact with the silver substrates of nanocrystals in the reaction mixture.
The Raman band at 1000 cm1 in the SERS spectra is caused by the ring breathing mode. When contrasted to the pristine Raman spectra, the spectrum was significantly moved to a higher frequencies area.
The TEM pictures show that the nanoparticles have a core-shell structure with a shadowy comparison for the Ag core with an 80 nm diameter and a bright contrast for the PLL shell with a homogenous thickness of 4 1 nm, meaning that the PLL coating of the Ag nanoparticles was effective.
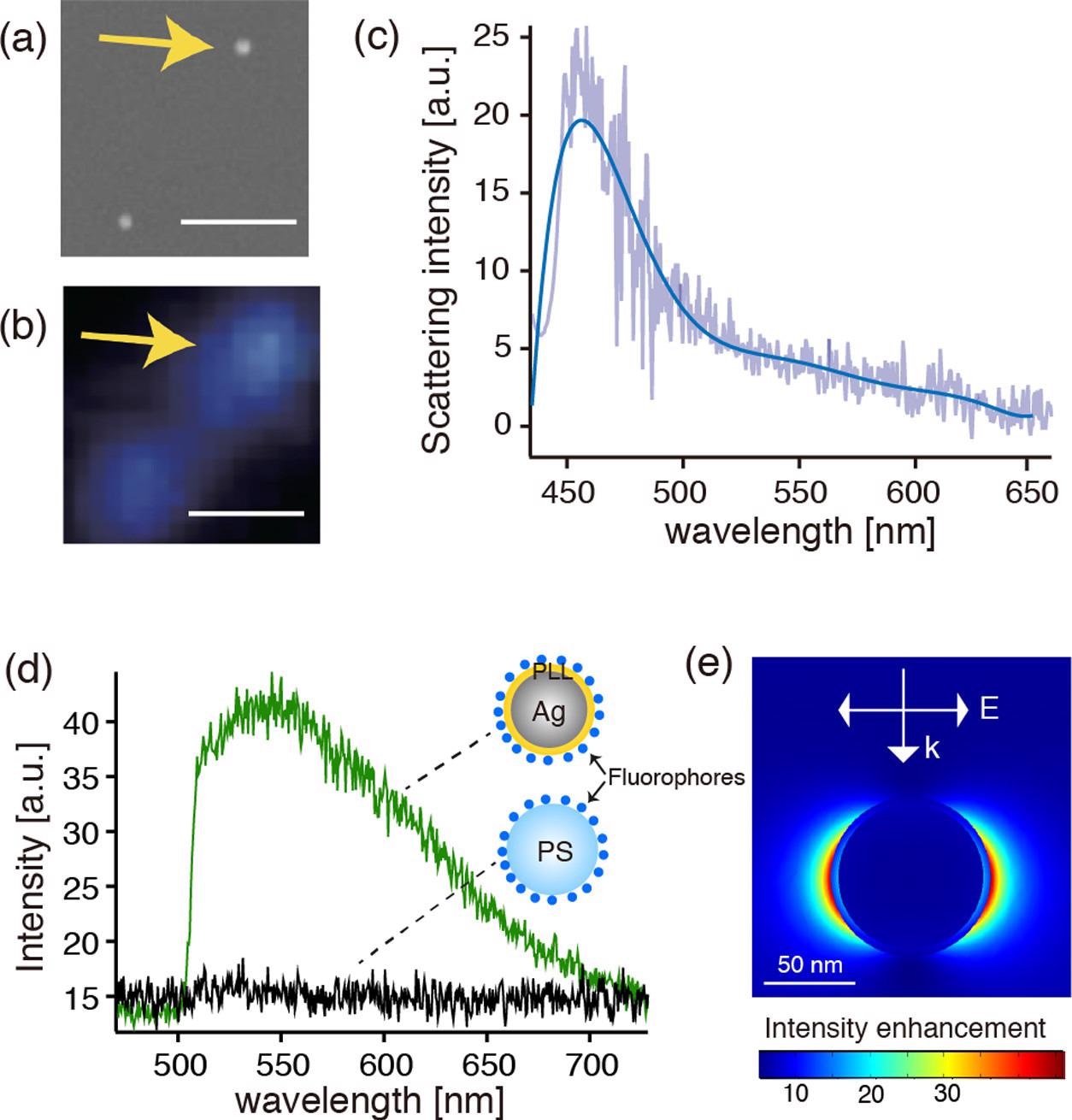
(a, b) SEM and dark-field optical images of the fluorophore-conjugated Ag@PLL nanoparticles dispersed on the glass substrate, respectively. The scale bars represent 1 μm. (c) Dark-field scattering spectrum of the single Ag nanoparticle indicated by the arrows in panels (a) and (b). (d) Fluorescence spectra measured on the Ag@PLL nanoparticle and the PS nanoparticles. (e) Calculated electric field distribution in the vicinity of a PLL-coated core–shell nanoparticle with a shell thickness of 4 nm and a core diameter of 80 nm, respectively, when illuminated by a plane wave of 450 nm. © Kato, R. et al., (2022)
When Ag nanoparticles were combined with PLL without the SPDP linker conjugation, a considerable increase in size and expanded dispersion was found, indicating the creation of additional nanocrystals.
The fluorescent transmitter with the PLL-coated Ag thin coatings was quelled down to 20% but was still perceptible which was caused by mechanical passivating inhibition. In contrast, the fluorescence intensity with the cysteamine-coated Ag film quelled more completely due to non-spontaneous energy transfer, which agreed with the previously reported distance reliance of fluorescence quenching in plasmon resonance.
To summarize, the disulfides were critical in covalently attaching PLL polymers to the interface of the Ag nanoparticles. Because of the covalently bonded link, the PLL coating was able to build a long-lasting stable layer on the Ag nanoparticles.
The coupling of the particle monitoring system in cells with the developed polymer-coated silver nanoparticles with fluorophores will open the path for high temporal and spatial precision investigation of biological processes.
Continue reading: New Method of 3D Plasmon Tomography.
Reference
Kato, R. et al., (2022) Highly Stable Polymer Coating on Silver Nanoparticles for Efficient Plasmonic Enhancement of Fluorescence. ACS Omega. Available at: https://pubs.acs.org/doi/10.1021/acsomega.1c06010
Disclaimer: The views expressed here are those of the author expressed in their private capacity and do not necessarily represent the views of AZoM.com Limited T/A AZoNetwork the owner and operator of this website. This disclaimer forms part of the Terms and conditions of use of this website.