The semiconductor industry is crucial for global technological advancement, but its rapid growth raises environmental concerns. From energy-intensive manufacturing to greenhouse gas emissions, semiconductors contribute substantially to climate change. However, in recent years, leading chipmakers have embraced sustainability through various technologies and approaches.
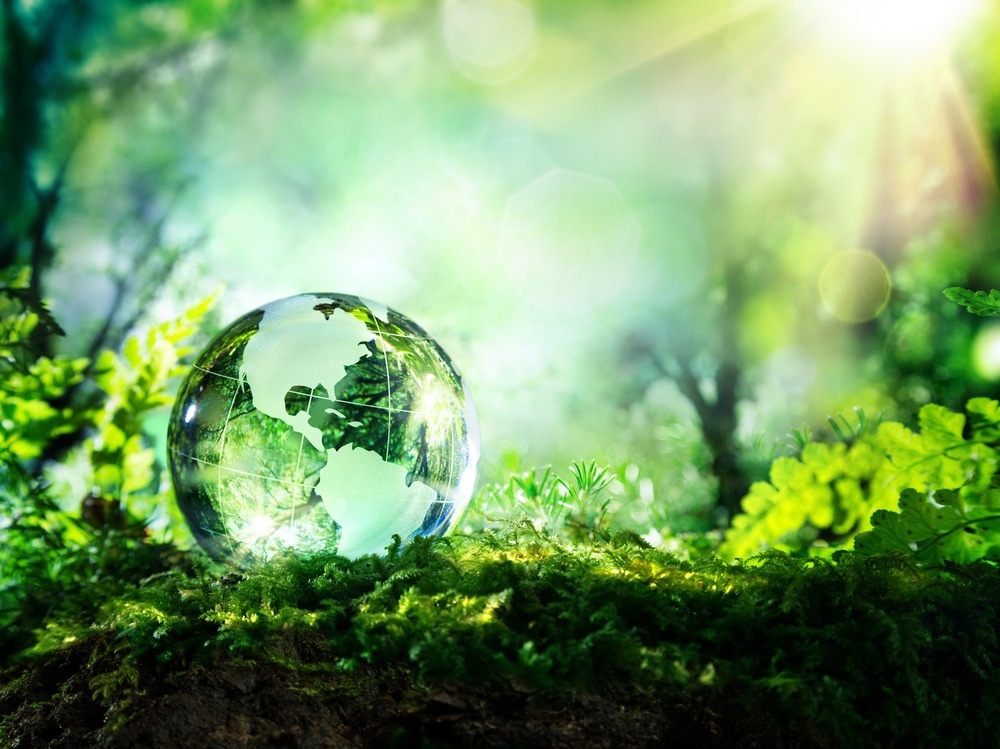
Image Credit: Romolo Tavani/Shutterstock.com
Semiconductor Industry Environmental Challenges
Semiconductor fabrication facilities (fabs) are extremely energy-intensive, producing high greenhouse gas emissions. Moreover, chip production requires vast amounts of ultra-pure water – a single facility can use over 10 million gallons per day, equal to a small city's consumption. This extensive water usage is especially concerning as many fabs are in water-stressed regions.
The complex chip manufacturing process involves over 400 hazardous chemicals and gases, producing substantial toxic waste. In addition, the industry also generates large volumes of electronic waste from discarded chips and components.
However, the industry is now taking steps to mitigate these challenges through renewable energy procurement, resource optimization, materials innovation, and circular economy approaches.
Transitioning to Renewable Energy
One of the biggest steps towards sustainability is switching to renewable energy sources, as semiconductor fabrication facilities are some of the most power-hungry manufacturing facilities in the world. For example, TSMC, the world's largest semiconductor chipmaker, uses more than 7% of Taiwan's electricity. Therefore, chipmakers can significantly reduce carbon emissions by tapping into clean energy like solar and wind instead of fossil fuels.
In 2020, TSMC became the first semiconductor company to join the RE100 initiative, committing to 100% renewable electricity by 2050. TSMC recently announced its largest renewable energy deal - a 20-year agreement to purchase 1 TWh per year from solar provider ARK Power. This is expected to reduce CO2 emissions by 500,000 metric tons annually.
Samsung has been running its US and China fabs on renewable energy since 2019, substantially reducing 5.68 million tons of greenhouse gases within the following year. These efforts demonstrate the industry's commitment to procuring more renewable electricity.
Developing Emissions Tracking Tools
While the complete global transition to renewable energy may take time, it is crucial to precisely measure emissions throughout the semiconductor supply chain to mitigate their environmental impact.
Imec, a Belgium-based R&D hub, has developed an innovative virtual fab model to assess the carbon footprint of chip production processes. This unique tool demonstrated that lithography and etching processes (semiconductor fabrication methods) account for 45% of Scope 1 and Scope 2 emissions while manufacturing advanced 3nm semiconductor chips.
The virtual fab can also precisely quantify emissions reduction from process optimizations like lowering extreme ultraviolet (EUV) scanner dosage.
Such granular data enables chipmakers to identify high-impact areas and implement targeted strategies to reduce emissions, energy, and resource usage. The virtual fab essentially acts as a digital twin to gauge sustainability parameters and compare process changes without disrupting real production lines.
Imec has also collaborated with Edwards Vacuum to install hydrogen recovery systems for EUV lithography processes in its advanced 300mm wafer fab. This system recovers and recycles over 70% of the hydrogen used during EUV manufacturing.
Adopting Circular Economy Principles: Waste Reduction and Recycling
Leading semiconductor chipmakers have embraced the circular economy concept, which focuses on recycling and reusing materials in a closed loop. With chip production requiring many scarce natural resources, reusing materials can significantly lower environmental impact.
Since 2015, TSMC has actively invested in developing reclaimed water technology, successfully repurposing 42.3 million tons of industrial reclaimed water in 2019, amounting to an impressive 67% of its total water consumption. Similarly, Dell contributes to water conservation by reusing wastewater from semiconductor chip manufacturing for landscape irrigation at its facility in China.
Intel has instituted solvent recovery systems to collect, clean and reuse key chemicals in manufacturing instead of disposing of them. In 2021, over 8,000 metric tons of the solvent were reclaimed and resold. The company also redesigned the packaging to enable box reuse, avoiding over 180 tons of material demand annually.
These efforts generated over $100 million in revenue, saved over $1 billion in costs, and facilitated the avoidance, recycling, reusing, or recovery of more than 130,000 metric tons of manufacturing waste.
Advanced semiconductor materials lithography's (ASML) Return4Reuse program aims to repurpose old equipment for less complex chip production, promoting sustainable material use throughout the value chain and processes by refurbished products. This initiative reduced total waste generated per €1 million, decreased from 417 kg to 315 kg, and generated $0.8 billion from reused parts.
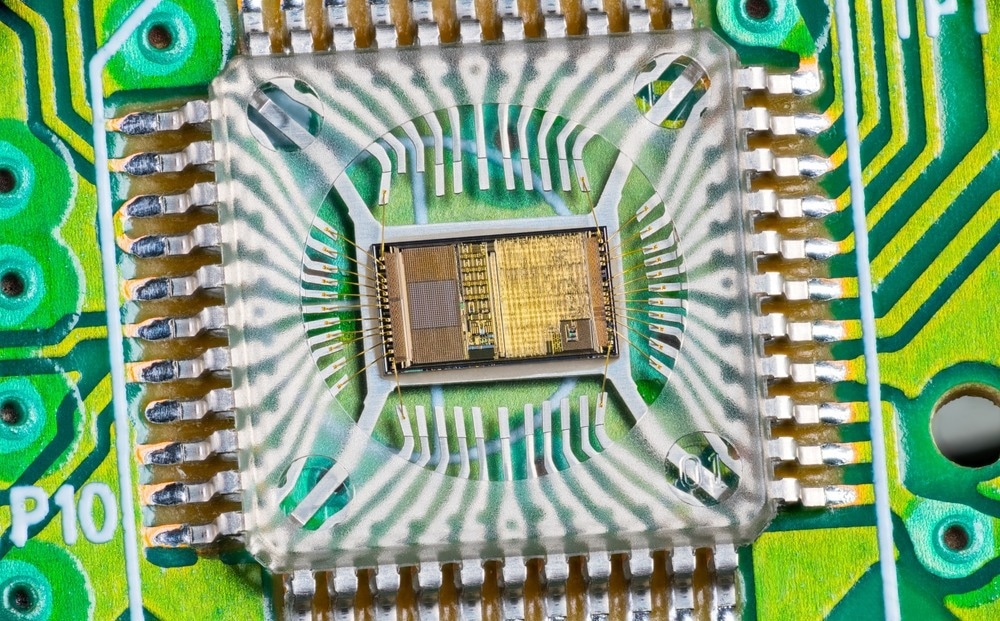
Image Credit: KPixMining/Shutterstock.com
Developing Alternative Sustainable Materials
Another key approach to reducing semiconductor's environmental impact is finding more sustainable alternatives to traditional materials like high-purity silicon, gallium, tungsten, and tantalum. The global depletion of these materials raises concerns about economic viability, making the adoption of greener substitutes imperative for a sustainable future in semiconductor manufacturing.
An MIT study demonstrated that cubic boron arsenide could be a game-changing semiconductor material. Due to its high thermal conductivity and electron-hole mobility, it is being hailed as potentially the most ideal semiconductor material ever discovered.
Additionally, graphene exhibits tremendous potential as a next-generation semiconductor material due to its exceptional properties, including extraordinarily high electron mobility (up to 250 times that of silicon), low loss requirements, small scale, and flexibility.
Other materials like gallium nitride, silicon carbide, molybdenum disulfide, and carbon nanotubes are under active investigation for their potential to outperform silicon, offering faster, more powerful, and energy-efficient semiconductor components.
Partnering Across the Supply Chain
Finally, coordination across the semiconductor supply chain is key to scale sustainability initiatives. That is why SEMI's (the global association for the semiconductor industry) sustainability advisory council is exploring ways to standardize sustainability reporting processes, ensuring accurate data sharing across the supply chain.
Semiconductor companies should also pursue responsible sourcing with their suppliers. For example, ASML adopts the Responsible Business Alliance (RBA) code of conduct and encourages key suppliers to do the same, fostering sustainability strategies.
Another effective supply chain strategy involves improving reverse logistics—repair, refurbishment, repackaging, recycling, and material harvesting—to reduce environmental, social, and economic impact.
The Path Forward
The semiconductor industry is still in the early stages of aligning on sustainability benchmarks and policy frameworks. However, promising solutions like renewables, alternative materials, resource recycling, and digitization will be essential to meet decarbonization goals.
With environmental regulations also set to tighten worldwide, sustainable technologies will become decisive competitive advantages. They benefit companies through energy and resource savings, future-proofing against climate risks, and meeting customer demands.
While the road ahead has obstacles, semiconductor companies are demonstrating climate solutions and innovation can advance together. The transition to a sustainable digital economy depends on their continued commitment to deploying cutting-edge green technologies.
References and Further Reading
Alam, S. (2022). 4 Strategies for Sustainable Semiconductor Manufacturing. [Online]. Available at: https://www.eetimes.com/4-strategies-for-sustainable-semiconductor-manufacturing/
ASML. (2023). Circular economy - Minimizing waste, maximizing resources. [Online]. Available at: https://www.asml.com/en/company/sustainability/circular-economy
Batra, G., Santhanam, N., &Surana, K. (2018). Graphene: The next S-curve for semiconductors? [Online]. Available at: https://www.mckinsey.com/industries/semiconductors/our-insights/graphene-the-next-s-curve-for-semiconductors
Brucker, V.M., & Jahn F. (2023). Sustainability in the semiconductor industry. [Online]. Available at: https://www.knowledgeagent.de/blog/posts/sustainability-in-the-semiconductor-industry/
David L. Chandler. (2022). The best semiconductor of them all? [Online]. Available at: https://news.mit.edu/2022/best-semiconductor-them-all-0721
DGB Group. (2023). Semiconductor sustainability: Paving the way for a greener technology industry. [Online]. Available at: https://www.green.earth/blog/semiconductor-sustainability-paving-the-way-for-a-greener-technology-industry
Gan, C. L., Chung, M. H., Zou, Y. S., Huang, C. Y., & Takiar, H. (2023). Technological Sustainable Materials and Enabling in Semiconductor Memory Industry: A Review. e-Prime-Advances in Electrical Engineering, Electronics and Energy, 100245. https://doi.org/10.1016/j.prime.2023.100245
Göke, S., Issler, M., Liu, D., Patel, M., and Spiller, P. (2023). Keeping the semiconductor industry on the path to net zero. [Online]. Available at: https://www.mckinsey.com/industries/semiconductors/our-insights/keeping-the-semiconductor-industry-on-the-path-to-net-zero
Intel. (2023). How Intel Supports a Circular Economy [Online]. Available at: https://www.intel.com/content/www/us/en/newsroom/news/how-intel-supports-circular-economy.html#gs.05rj2e
JLL. (2023). How semiconductor firms are chipping in on sustainability. [Online]. Available at: https://www.us.jll.com/en/trends-and-insights/cities/how-semiconductor-firms-are-chipping-in-on-sustainability
Johnson, J. (2022). Scarcity Drives Fabs to Wastewater Recycling. [Online]. Available at: https://spectrum.ieee.org/fabs-cut-back-water-use
Mann, E. (2023). Balancing semiconductor innovation vs sustainability. [Online]. Available at: https://electronics360.globalspec.com/article/20060/balancing-semiconductor-innovation-vs-sustainability
Photonics. (2023). Virtual Semiconductor Fab Reduces Manufacturing Carbon Footprint. [Online]. Available at: https://www.photonics.com/Articles/Virtual_Semiconductor_Fab_Reduces_Manufacturing/a68825
Samsung. (2022). Samsung Electronics Announces New Environmental Strategy. [Online]. Available at: https://news.samsung.com/global/samsung-electronics-announces-new-environmental-strategy
Shin, J., Gamage, G. A., Ding, Z., Chen, K., Tian, F., Qian, X., ... & Chen, G. (2022). High ambipolar mobility in cubic boron arsenide. Science, 377(6604), 437-440. https://doi.org/10.1126/science.abn4290
Wang, D., Wang, L., & Syu F-S. (2023). TSMC Pioneers Joint Procurement of Renewable Energy to Strengthen Suppliers' Carbon Reduction Capabilities. [Online]. Available at: https://esg.tsmc.com/en/update/greenManufacturing/caseStudy/69/index.html
Disclaimer: The views expressed here are those of the author expressed in their private capacity and do not necessarily represent the views of AZoM.com Limited T/A AZoNetwork the owner and operator of this website. This disclaimer forms part of the Terms and conditions of use of this website.